Artificial light at night (ALAN) has become a worldwide concern, with the American Medical Association declaring it a form of pollution. Birds’ daily physiology and behavior are disrupted by ALAN exposure, and nighttime melatonin synthesis is suppressed. This article will summarize a recent study published in the journal Birds looking at this further within songbirds.
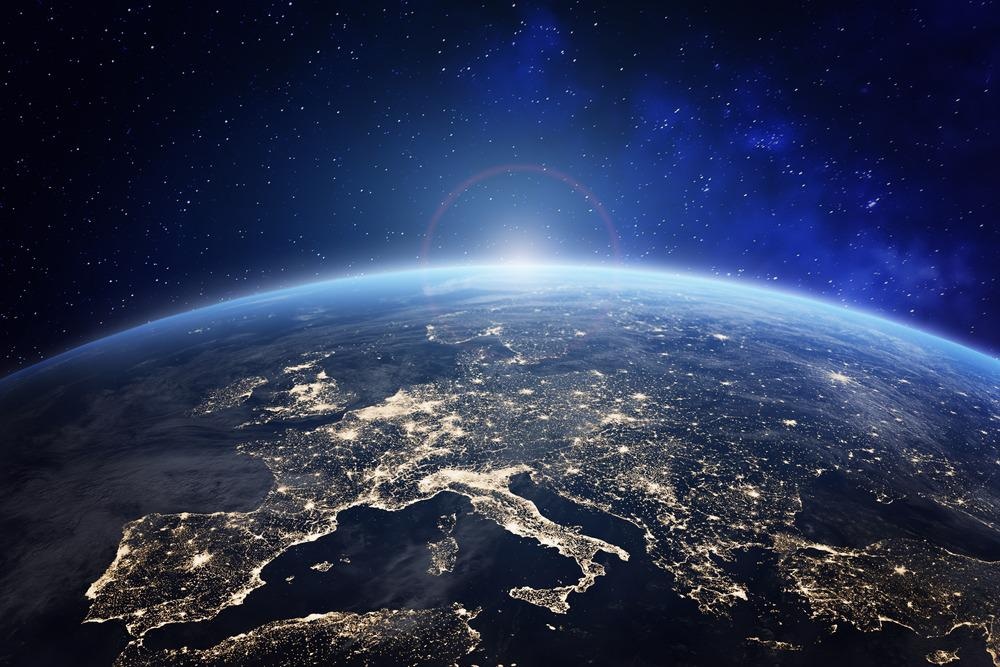
Image Credit: NicoElNino/Shutterstock.com
In both birds and mammals, ALAN exposure has been linked to major ecological and physiological implications, including impairment of immunological, metabolic, reproductive, and cognitive processes.
The presence of ALAN disrupts the natural habitat, with bright intensity of light close to the light source and low intensity of light at long distances at night. Evidence shows that both no-night (24-hour constant light, LL) and dLAN (dim light at night) have comparable effects in laboratory experiments.
ALAN has negative impacts on various territorial behaviors in wild birds, including reproduction, singing, migration, and sleep. ALAN (LL and dLAN) also has detrimental impacts on learning, memory, mood, and exploration in multiple laboratory investigations.
Melatonin and epigenetic alterations may have a role in ALAN-induced reactions, according to the findings. The underlying mechanism(s) are generic and applicable to a wide range of species; nevertheless, for a better understanding of the issue, the study concentrated on bird species and addressed the relevance of the avian system.
Methodology
Google Scholar was used to find research publications and reports from 2006 to 2021. The search keywords “light at night + sleep + cognition + brain function,” as well as terms and phrases linked with “neuron,” such as “light at night + neurogenesis” or “light at night + sleep + cognition + brain function + neurotransmitter,” were utilized.
Adding the phrases “light at night + sleep + cognition + neuron + melatonin + birds” to the search criteria resulted in the discovery of 3420 publications and papers. The abstracts or full texts of these publications were analyzed to find articles or reports from research on bird species.
Papers that offered no new evidence linked to avian behavioral changes under illumination at night or merely presented viewpoints or opinions were excluded.
Results
House Crows subjected to dLAN exhibited depressive-like behaviors such as lower feeding and grooming, as well as increased feather-picking and self-mutilation consistent with sleep deprivation, according to research. dLAN also decreased neurogenesis in the hippocampus and impacted the expression of numerous genes.
In rodents, sleep deprivation is linked to memory loss, impaired attention and decision-making, and mood abnormalities. ALAN-induced sleep impairments and cognitive dysfunctions in House Crows and Zebra Finches have been seen in birds.
Peafowl (Pavo cristatus) and Great Tits exposed to ALAN, on the other hand, demonstrated sleep disturbance but no cognitive impairment. ALAN-induced depressed behavior was seen in nocturnal mice in a few trials, although there were no effects on sleep following ALAN administration.
Table 1 summarizes the findings of current research on several bird species. The extent of the link between sleep and cognitive functioning, on the other hand, is unknown, and further study is needed. However, as previously mentioned, the effects of ALAN on brain functioning may also be mediated through the hormonal system.
Table 1. Summary of the results from recent studies in different avian species highlighting the effects of light at night on behavioral phenotypes and the molecular correlates. Source: Taufique, 2022
Species |
Light
Environment |
Affected Behavioural Phenotype |
Molecular
Correlates |
Study |
American Robins (Turdus migratorius) |
ALAN |
Advances the morning chorus into the night |
|
Miller [23] |
European Robin (Erithacus rubecula)
Eurasian Blackbird (Turdus merula)
Song Thrush (Turdus philomelos)
Great Tits (Parus major)
Blue Tits (Cyanistes caeruleus)
Common Chaffinch (Fringilla coelebs) |
dLAN |
Alters the phenology of dawn and dusk singing (Earlier in the year than before) |
|
Da Silva et al. [8] |
Blackbirds |
dLAN (~0.3 lux) |
Early-onset of activity and night restlessness |
|
Dominoni and Partecke [22] |
Blackbirds |
dLAN (~0.3 lux) |
Increase in pre-dawn activity |
Decreased nocturnal melatonin levels |
Dominoni et al. [54] |
Blackbirds |
dLAN (~0.3 lux) |
Advances seasonal testicular growth. |
|
Dominoni et al. [55] |
Great Tits |
dLAN (~ 1.6 lux) |
Sleep deficits |
|
Raap et al. [10] |
Great Tits |
Different doses of dLAN (~0.05, 0.15, 0.5, 1.5 or 5 lux) |
Dose-dependent increase in nocturnal activity |
Dose-dependent reduction in nocturnal melatonin levels |
deJong et al. [23] |
Great Tits |
dLAN (~8 lux) of different wavelength |
Increase in nocturnal activity
Sleep deficits |
Decreased plasma oxalic acid
Reduced telomere length (cellular aging) |
Ouyang et al. [11] |
Great Tits |
dLAN (~0.1, 0.5, 1.5 and 5 lux) |
Advanced wake-up time |
Shift in bmal1 expression
Shift in metabolite expressions
Desynchronization of metabolic and immune genes |
Dominoni et al. [25] |
Blue Tits
(Cyanistes caeruleus) |
dLAN (2 lux) |
|
Affects feather glucocorticoid levels |
Dominoni et al. [56] |
Indian Peafowl (Pavo cristatus) |
dLAN (~0.75 lux) |
Increased nocturnal vigilance
Sleep loss |
|
Yorzinski et al. [33] |
European Nightjars (Caprimulgus europaeus) |
dLAN |
Increased foraging opportunity
Changes in habitat selection |
|
Sierro and Erhardt [15] |
Burrowing Owl (Athene cunicularia) |
dLAN |
Increased foraging opportunity
Nest habitat selection near light source |
|
Rodríguez et al. [16] |
House Crow (Corvus splendens) |
LL (~150 lux) |
Activity rhythm disruption
Learning and memory deficits |
Reduced neuronal activity in HP and NC
Decreased expression of tyrosine hydroxylase in the mid-brain |
Taufique and Kumar [17] |
House Crow |
LL (~150 lux) |
Learning and memory deficits |
Decreased neurogenesis and dendritic complexity in HP and NC |
Taufique et al. [36] |
House Crow |
dLAN (~6 lux) |
Increase in nocturnal activity
Sleep deprivation
Depressive-like |
Decreased expression of BDNF, IL1β, TNFR1, NR4A2 in HPs
Increased HDAC4 expression and histone H3 acetylation of BDNF gene in HP
Decreased neurogenesis in HP
Decreased levels of nocturnal melatonin |
Taufique et al. [18]
* Behavioural phenotypes were rescued by elimination of dLAN |
House Crow |
LL (~150 lux) and dLAN (~6 lux) |
|
Decreased neuronal soma size
Reduced glia–neuron ratio |
Taufique et al. [42] |
Zebra Finch
(Taeniopygia guttata) |
LL (~5 lux) |
Disturbed activity rhythm
Learning deficits |
|
Jha and Kumar [19] |
Zebra Finch (male) |
LL (~150 lux) |
Disrupted activity and singing behavior (30% of individuals)
Decline in song quality, reduced amplitude and song production |
Loss of rhythm in the expression of clock genes in the hypothalamus |
Prabhat et al. [27] |
Zebra Finch (female) |
LL (~150 lux) |
Fattening, weight gain, and lipid accumulation in the liver |
Loss of rhythm in the expression of clock genes in the hypothalamus and peripheral tissues |
Prabhat et al. [28] |
Zebra Finch |
LL (~400 lux) and dLAN (~3 lux) |
|
Loss of melatonin and corticosterone diurnal pattern
Altered diurnal pattern of cytokines in the brain |
Mishra et al. [31] |
Zebra Finch |
dLAN (~5 lux) |
Induced night-time feeding and perch-hopping
Sleep deprivation
Learning and memory deficits
Increased neophobia |
|
Prabhat et al. [37] |
Zebra Finch |
dLAN (~5 lux) |
Sleep deficits |
Decreased plasma oxalate levels
Decreased tlr4, il-b, nos gene expression, and attenuated achm3 mRNA levels
Changes in gene expression of Ca2+ dependent sleep-inducing pathway |
Batra et al. [26] |
Zebra Finch |
dLAN (~5 lux) |
Increased night-time activity
Nocturnal feeding
Body fattening and weight gain |
Increased levels of plasma glucose
Decreased levels of thyroxine and triglycerides
Altered metabolic genes expression (sirt1, g6pc, and foxo1) |
Batra et al. [29] |
Zebra Finch |
LL (~150 lux) and dLAN (~5 lux) |
Body fattening and weight gain
Hepatic lipid accumulation |
Changes in gut microbiome with a decline in Lactobacillus richness |
Malik et al. [30]
* Phenotype was rescued by Lactobacillus supplement |
Zebra Finch |
dLAN |
|
Increased neuronal recruitment reduced neuronal density in the hippocampus
Decreased nocturnal melatonin levels |
Moaraf et al. [40,41] |
Domestic Pigeons (Columba livia domestica) and Australian Magpies (Cracticus tibicen tyrannica) |
dLAN (~9.6 and 18.89) |
Reduced sleep duration and fragmentation
Slow-wave activity during non-REM sleep
Reduced REM sleep |
|
Aulsebrook et al. [34] |
Melatonin, which is generated by the pineal gland and released into the third ventricle, is involved in a variety of biological activities and communicates ambient light-dark information to the brain. Melatonin modulates memory and cognition via the MT1 and MT2 receptors.
Melatonin is generated in both nocturnal and diurnal animals during the dark period of the night. However, mice strains from the laboratory have no discernible melatonin rhythms. Melatonin synthesis is suppressed in diurnal species, including humans, when they are exposed to low-intensity light.
dLAN circumstances have been shown to decrease melatonin and impact neurogenesis in diurnal birds, according to publications. Tree Sparrows from urban areas have been shown to have lower plasma melatonin levels than those from rural areas. The findings showed that in urban Tree Sparrows, expression of the Aanat, Mel1, and Mel2 genes in the pineal is altered.
The control of different processes, including brain functioning and mental health, is aided by epigenetic alterations of gene expression. Melatonin has been reported to affect epigenetic medicines and gene expressions, suggesting that it might play a key function in epigenetic control.
Conclusion
The extensive use of electrical lighting, as well as the resulting light pollution, is affecting the temporal arrangement of biological activity in animals, such as sleep and cognitive functioning.
Considering the evidence linking disturbed circadian processes to poor cognitive function, ALAN might be contributing to learning and memory problems as well as depression in people via a variety of circadian controlled pathways, as illustrated in Figure 1.
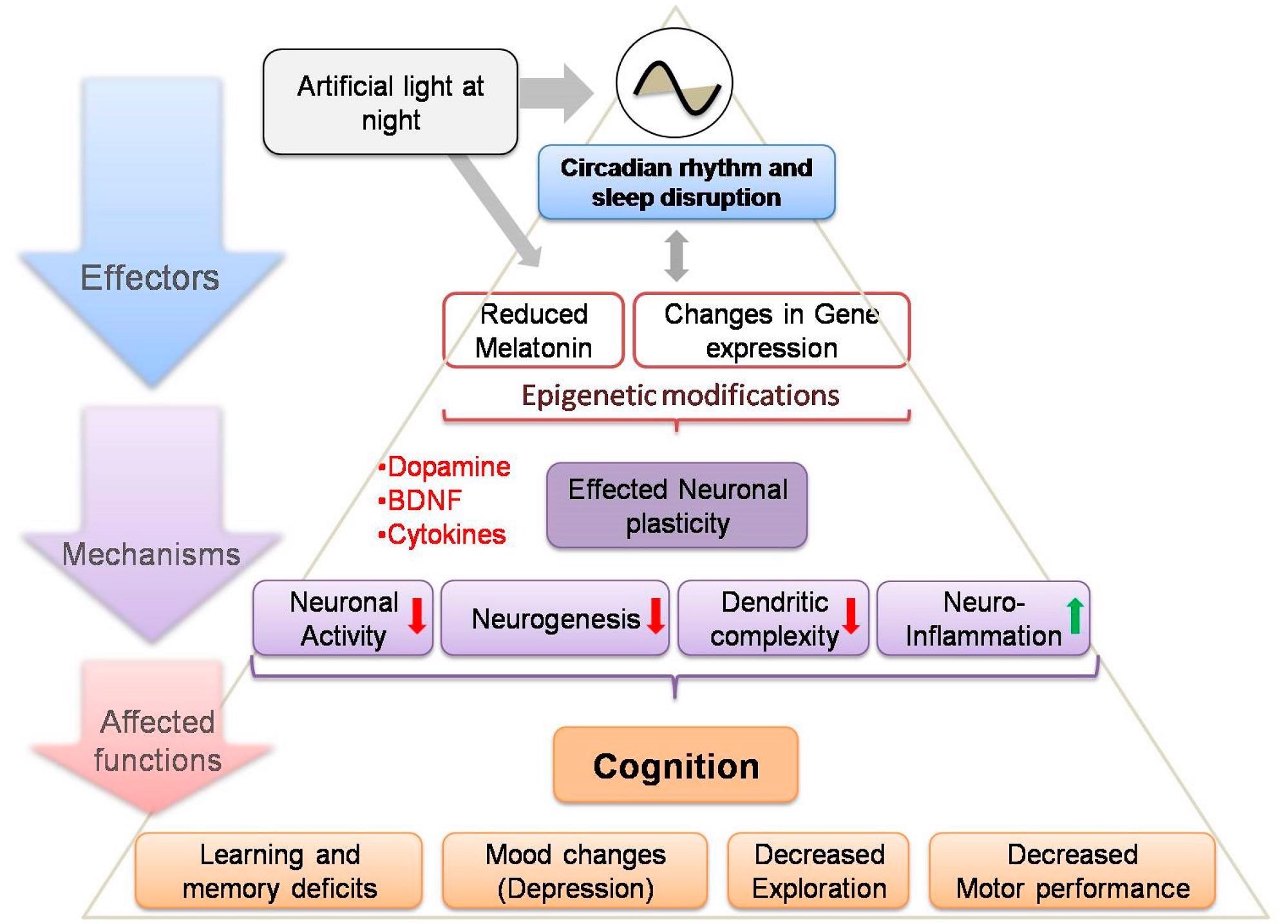
Figure 1. Schematic depicting effect of ALAN exposure, acting on different levels of organization (affecters) via a number of neuronal pathways (mechanisms), those resulting in a series of adverse effects on brain functions (affected functions). This model may help us to understand the complexity of the relationships among exposure components in a systematic manner and may help in assessing the impact on brain functions of ALAN exposure outcomes and their underlying mechanisms (red and green arrows indicate decreased and increased functions, respectively). BDNF: Brain-derived neurotropic factor. Image Credit: Taufique, 2022
Melatonin may serve as a connection between environmental disturbance of biological cycles and epigenetic changes in genes involved in brain function control.
Zebra Finches would be a good model for these objectives since they reproduce in labs and have been utilized in numerous researches to better understand avian biology, despite being an oddity among passerine birds.
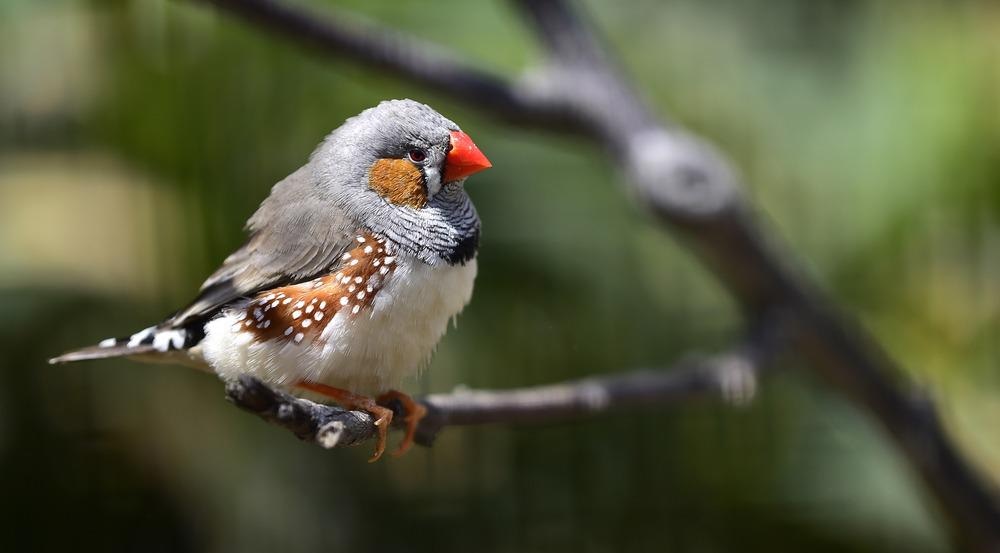
Zebra finch. Image Credit: Wang LiQiang/Shutterstock.com
Despite the substantial prevalence of artificial light at night across the world, it is critical to understand the impacts of ALAN on bird physiologies and behaviors, as well as to identify a safe distance from light sources to reduce the concerns.
Due to the small number of research that has been undertaken, which is also confined to a few species and/or short-term trials, determining the influence on a population is challenging. Understanding the long-term processes that alter communities and ecosystems is crucial to predicting the ecological implications of LAN.
It is also crucial to set out repeatable field and laboratory trials to see how LAN affects populations, communities, and ecosystems.
Consequently, it is critical to focus current research on effective mitigation measures to balance the human demand for illumination in society with the needs of other animals for darkness.
Small but significant global conservation efforts, such as the Fatal Light Awareness Program (FLAP) in Canada and the Lights Out Program by the Audubon Society in the United States, could help manage the negative effects of LAN by advocating the importance of darker nights. Curbing light pollution and limiting the problem can be beneficial for all species worldwide.
The majority of the information on the negative effects of LAN on cognitive functioning comes from nocturnal rodent research, with a few from diurnal species, as previously indicated.
Additional research on diurnal species, such as birds, should be conducted to better duplicate human exposure and its effects, as well as to clarify the mechanism(s) through which LAN exposures result in deleterious outcomes.
Journal Reference
Taufique, S. K. T. (2022) Artificial Light at Night, Higher Brain Functions and Associated Neuronal Changes: An Avian Perspective. Birds, 3(1), pp. 38–50 Available Online: https://www.mdpi.com/2673-6004/3/1/3/htm
References and Further Reading
- Falchi, F., et al. (2016) The new world atlas of artificial night sky brightness. Science Advances, 2, p. e1600377. doi.org/10.1126/sciadv.1600377.
- Rich, C & Longcore, T (2006) Ecological Consequences of Artificial Night Lighting; Island Press: Washington, DC, USA.
- Navara, K J & Nelson, R J (2007) The dark side of light at night: Physiological, epidemiological, and ecological consequences. Journal of Pineal Research, 43, pp. 215–224. doi.org/10.1111/j.1600-079X.2007.00473.x.
- Gaston, K. J., et al. (2017) Impacts of Artificial Light at Night on Biological Timings. Annual Review of Ecology, Evolution, and Systematics, 48, pp. 49–68. doi.org/10.1146/annurev-ecolsys-110316-022745.
- Dominoni, D. M., et al. (2016) Light at night, clocks and health: From humans to wild organisms. Biology Letters, 12, p. 20160015. doi.org/10.1098/rsbl.2016.0015.
- Lunn, R. M., et al. (2017) Health consequences of electric lighting practices in the modern world: A report on the National Toxicology Program’s workshop on shift work at night, artificial light at night, and circadian disruption. Science of The Total Environment, 607, pp. 1073–1084. doi.org/10.1016/j.scitotenv.2017.07.056.
- Kempenaers, B., et al. (2010) Artificial night lighting affects dawn song, extra-pair siring success, and lay date in songbirds. Current Biology, 20, pp. 1735–1739. doi.org/10.1016/j.cub.2010.08.028.
- Da Silva, A., et al. (2015) Light pollution alters the phenology of dawn and dusk singing in common European songbirds. Philosophical Transactions of the Royal Society B, 370, p. 20140126. doi.org/10.1098/rstb.2014.0126.
- McLaren, J. D., et al. (2018) Artificial light at night confounds broad-scale habitat use by migrating birds. Ecology Letters, 21, pp. 356–364. doi.org/10.1111/ele.12902.
- Raap, T., et al. (2016) Artificial light at night disrupts sleep in female great tits (Parus major) during the nestling period, and is followed by a sleep rebound. Environmental Pollution, 215, pp. 125–134. doi.org/10.1016/j.envpol.2016.04.100.
- Ouyang, J. Q., et al. (2017) Restless roosts: Light pollution affects behavior, sleep, and physiology in a freeliving songbird. Global Change Biology, 23, pp. 4987–4994. doi.org/10.1111/gcb.13756.
- Leveau, L M (2020) Artificial Light at Night (ALAN) Is the Main Driver of Nocturnal Feral Pigeon (Columba livia f. domestica) Foraging in Urban Areas. Animals, 10, p. 554. doi.org/10.3390/ani10040554.
- Lourenço, P M (2012) Southern Lapwings Vanelluschilensis may take advantage of artificial illumination duringnight foraging. Wader Study Group Bull, 119, p. 61.
- Stracey, C. M., et al. (2014) Light pollution allows the northern mockingbird (Mimuspolyglottos) to feed nestlings after dark. The Wilson Journal of Ornithology, 126, pp. 366–369. doi.org/10.1676/13-107.1.
- Sierro, A & Erhardt, A (2020) Light pollution hampers recolonization of revitalised European Nightjar habitats in the Valais (Swiss Alps). Journal of Ornithology, 160, pp. 749–761. doi.org/10.1007/s10336-019-01659-6.
- Rodríguez, A., et al. (2020) Artificial light at night as a driver of urban colonization by an avian predator. Landscape Ecology, 36, pp. 17–27. doi.org/10.1007/s10980-020-01132-3.
- Taufique, S T & Kumar, V (2016) Differential activation and tyrosine hydroxylase distribution in the hippocampal, pallial and midbrain brain regions in response to cognitive performance in Indian house crows exposed to abrupt light environment. Behavioural Brain Research, 314, pp. 21–29. doi.org/10.1016/j.bbr.2016.07.046.
- Taufique, S. K. T., et al. (2018) Illuminated night alters hippocampal gene expressions and induces depressive-like responses in diurnal corvids. European Journal of Neuroscience, 48, pp. 3005–3018. doi.org/10.1111/ejn.14157.
- Jha, N A & Kumar, V (2017) Effect of no-night light environment on behaviour, learning performance and personality in zebra finches. Animal Behaviour, 132, pp. 29–47. doi.org/10.1016/j.anbehav.2017.07.017.
- Cassone, V M (2013) Avian circadian organization: A chorus of clocks. Frontiers in Neuroendocrinology, 35, pp. 76–88. doi.org/10.1016/j.yfrne.2013.10.002.
- Dominoni, D M & Partecke, J (2015) Does light pollution alter daylength? A test using light loggers on free-ranging European blackbirds (Turdusmerula). Philosophical Transactions of the Royal Society B: Biological Sciences, 370, p. 20140118. doi.org/10.1098/rstb.2014.0118.
- de Jong, M., et al. (2016) Dose-dependent responses of avian daily rhythms to artificial light at night. Physiology Behavior, 155, pp. 172–179. doi.org/10.1016/j.physbeh.2015.12.012.
- Miller, M W (2006) Apparent effects of light pollution on singing behavior of American robins. The Condor, 108, pp. 130–139. doi.org/10.1093/condor/108.1.130.
- Foster, R G & Kreitzman, L A (2005) Time to Reap. BioScience, 55, pp. 795–797.
- Dominoni, D. M., et al. (2021) Artificial light at night leads to circadian disruption in a songbird: Integrated evidence from behavioural, genomic and metabolomic data. bioRxiv. doi.org/10.1101/2020.12.18.423473.
- Batra, T., et al. (2020) Sleep in unnatural times: Illuminated night negatively affects sleep and associated hypothalamic gene expressions in diurnal zebra finches. Proceedings of the Royal Society B: Biological Sciences, 287, p. 20192952. doi.org/10.1098/rspb.2019.2952.
- Prabhat, A., et al. (2019) Dissociation of circadian activity and singing behaviour from gene expression rhythms in the hypothalamus, song control nuclei and cerebellum in diurnal zebra finches. Chronobiology International, 36, pp. 1268–1284. doi.org/10.1080/07420528.2019.1637887.
- Prabhat, A., et al. (2020) Developmental effects of constant light on circadian behaviour and gene expressions in zebra finches: Insights into mechanisms of metabolic adaptation to aperiodic environment in diurnal animals. Journal of Photochemistry and Photobiology B: Biology, 211, p. 111995. doi.org/10.1016/j.jphotobiol.2020.111995.
- Batra, T., et al. (2019) Illuminated night alters behaviour and negatively affects physiology and metabolism in diurnal zebra finches. Environmental Pollution, 254, p. 112916. doi.org/10.1016/j.envpol.2019.07.084.
- Malik, I., et al. (2020) Light at night affects gut microbial community and negatively impacts host physiology in diurnal animals: Evidence from captive zebra finches. Microbiological Research, 241, p. 126597. doi.org/10.1016/j.micres.2020.126597.
- Mishra, I., et al. (2019) Light at night disrupts diel patterns of cytokine gene expression and endocrine profiles in zebra finch (Taeniopygia guttata). Scientific Reports, 9, p. 15833. doi.org/10.1038/s41598-019-51791-9.
- Renthlei, Z & Trivedi, A K (2019) Effect of urban environment on pineal machinery and clock genes expression of tree sparrow (Passer montanus). Environmental Pollution, 255, p. 113278. doi.org/10.1016/j.envpol.2019.113278.
- Yorzinski, J. L., et al. (2015) Artificial light pollution increases nocturnal vigilance in peahens. PeerJ, 3, p. e1174. doi.org/10.7717/peerj.1174.
- Aulsebrook, A. E., et al. (2020) White and Amber Light at Night Disrupt Sleep Physiology in Birds. Current Biology, 30, pp. 3657–3663.e5. doi.org/10.1016/j.cub.2020.06.085.
- Romeo, S., et al. (2013) Bright light exposure reduces TH-positive dopamine neurons: Implications of light pollution in Parkinson’s disease epidemiology. Scientific Reports, 3, p. 1395. doi.org/10.1038/srep01395.
- Taufique, S. T., et al. (2018) Constant light environment suppresses maturation and reduces complexity of new born neuron processes in the hippocampus and caudal nidopallium of a diurnal corvid: Implication for impairment of the learning and cognitive performance. Neurobiology of Learning and Memory, 147, pp. 120–127. doi.org/10.1016/j.nlm.2017.12.001.
- Prabhat, A., et al. (2021) Effects of Night Illumination on Behavior, Body Mass and Learning in Male Zebra Finches. Birds, 2, pp. 381–394. doi.org/10.3390/birds2040028.
- Bordnick, P. S., et al. (1994) Feather picking disorder and trichotillomania: An avian model of human psychopathology. Journal of Behavior Therapy and Experimental Psychiatry, 25, pp. 189–196. doi.org/10.1016/0005-7916(94)90019-1.
- Krishnan, V & Nestler, E J (2010) Linking Molecules to Mood: New Insight Into the Biology of Depression. The American Journal of Psychiatry, 167, pp. 1305–1320. doi.org/10.1176/appi.ajp.2009.10030434.
- Moaraf, S., et al. (2020) Artificial light at night affects brain plasticity and melatonin in birds. Neuroscience Letters, 716, p. 134639.
- Moaraf, S., et al. (2020) Artificial Light at Night Increases Recruitment of New Neurons and Differentially Affects Various Brain Regions in Female Zebra Finches. International Journal of Molecular Sciences, 21, p. 6140. doi.org/10.3390/ijms21176140.
- Taufique, S. T., et al. (2019) Light at night affects hippocampal and nidopallial cytoarchitecture: Implication for impairment of brain function in diurnal corvids. Journal of Experimental Zoology. Part A, Ecological and Integrative Physiology, 331, pp. 149–156. doi.org/10.1002/jez.2238.
- Sherwood, C. C., et al. (2006) Evolution of increased glia-neuron ratios in the human frontal cortex. Proceedings of the National Academy of Sciences of the United States of America. USA, 103, pp. 13606–13611. doi.org/10.1073/pnas.0605843103.
- Freas, C., et al. (2013) Hippocampal neuron soma size is associated with population differences in winter climate severity in food-caching chickadees. Functional Ecology, 27, pp. 1341–1349. doi.org/10.1111/1365-2435.12125.
- Herculano-Houzel, S (2014) The glia/neuron ratio: How it varies uniformly across brain structures and species and what that means for brain physiology and evolution. Glia, 62, pp. 1377–1391. doi.org/10.1002/glia.22683.
- Cotter, D., et al. (2001) Reduced Glial Cell Density and Neuronal Size in the Anterior Cingulate Cortex in Major Depressive Disorder. Arch Gen Psychiatry, 58, pp. 545–553. doi.org/10.1001/archpsyc.58.6.545.
- Stockmeier, C. A., et al. (2004) Cellular changes in the postmortem hippocampus in major depression. Biol. Psychiatry, 56, pp. 640–650. doi.org/10.1016/j.biopsych.2004.08.022.
- Ma, W. P., et al. (2007) Exposure to chronic constant light impairs spatial memory and influences long-term depression in rats. Neuroscience Research, 59, pp. 224–230. doi.org/10.1016/j.neures.2007.06.1474.
- Fujioka, A., et al. (2011) Effects of a constant light environment on hippocampal neurogenesis and memory in mice. Neuroscience Letters, 488, pp. 41–44. doi.org/10.1016/j.neulet.2010.11.001.
- Tsuno, N., et al. (2005) Sleep and depression. The Journal of Clinical Psychiatry, 66, pp. 1254–1269.
- Bedrosian, T A & Nelson, R J (2017) Timing of light exposure affects mood and brain circuits. Translational Psychiatry, 7, p. e1017. doi.org/10.1038/tp.2016.262.
- Ulgezen, Z. N., et al. (2019) The preference and costs of sleeping under light at night in forest and urban great tits. Proceedings of the Royal Society B: Biological Sciences, 286, p. 20190872. doi.org/10.1098/rspb.2019.0872.
- Borniger, J. C., et al. (2013) Dim Light at Night Does Not Disrupt Timing or Quality of Sleep in Mice. Chronobiology International, 30, pp. 1016–1023. doi.org/10.3109/07420528.2013.803196.
- Dominoni, D. M., et al. (2013) Urban-like night illumination reduces melatonin release in European blackbirds (Turdusmerula): Implications of city life for biological timekeeping of songbirds. Frontiers in Zoology, 10, p. 60. doi.org/10.1186/1742-9994-10-60
- Dominoni, D., et al. (2013) Artificial light at night advances avian reproductive physiology. Proceedings of the Royal Society B: Biological Sciences, 280, p. 20123017. doi.org/10.1098/rspb.2012.3017.
- Dominoni, D. M., et al. (2021) Feather, But Not Plasma, Glucocorticoid Response to Artificial Light at Night Differs between Urban and Forest Blue Tit Nestlings. Integrative and Comparative Biology (ICB), 61, pp. 1111–1121. doi.org/10.1093/icb/icab067.
- Reiter, R J (2003) Melatonin: Clinical relevance. Best Practice & Research Clinical Endocrinology & Metabolism, 17, pp. 273–285. doi.org/10.1016/S1521-690X(03)00016-2.
- Hardeland, R (2013) Chronobiology of Melatonin beyond the Feedback to the Suprachiasmatic Nucleus—Consequences to Melatonin Dysfunction. International Journal of Molecular Sciences, 14, pp. 5817–5841. doi.org/10.3390/ijms14035817.
- Liu, J., et al. (2017) Role of the MT1 and MT2 melatonin receptors in mediating depressive and anxiety-like behaviors in C3H/HeN mice. Genes Brain and Behavior, 16, pp. 546–553. doi.org/10.1111/gbb.12369.
- Ramirez-Rodriguez, G., et al. (2011) Chronic treatment with melatonin stimulates dendrite maturation and complexity in adult hippocampal neurogenesis of mice. Journal of Pineal Research, 50, pp. 29–37. doi.org/10.1111/j.1600-079X.2010.00802.x.
- Fredrich, M., et al. (2017) Impact of melatonin receptor-signaling on Zeitgeber time-dependent changes in cell proliferation and apoptosis in the adult murine hippocampus. Hippocampus, 27, pp. 495–506. doi.org/10.1002/hipo.22706.
- Alzoubi, K. H., et al. (2016) Chronic melatonin treat-ment prevents memory impairment induced by chronic sleep deprivation. Molecular Neurobiology, 53, pp. 3439–3447. doi.org/10.1007/s12035-015-9286-z.
- Zhang, L., et al. (2013) Melatonin ameliorates cognitive impairment induced by sleep deprivation in rats: Role of oxidative stress, BDNF and CaMKII. Behavioural Brain Research, 256, pp. 72–81. doi.org/10.1016/j.bbr.2013.07.051.
- Brüning, A., et al. (2015) Spotlight on fish: Light pollution affects circadian rhythms of European perch but does not cause stress. Science of The Total Environment, 511, pp. 516–522. doi.org/10.1016/j.scitotenv.2014.12.094.
- Brüning, A., et al. (2018) Influence of light intensity and spectral composition of artificial light at night on melatonin rhythm and mRNA expression of gonadotropins in roach Rutilusrutilus. Fish Physiology and Biochemistry, 44, pp. 1–12. doi.org/10.1007/s10695-017-0408-6.
- Cajochen, C., et al. (2005) High Sensitivity of Human Melatonin, Alertness, Thermoregulation, and Heart Rate to Short Wavelength Light. The Journal of Clinical Endocrinology & Metabolism, 90, pp. 1311–1316. doi.org/10.1210/jc.2004-0957.
- Sahar, S & Sassone-Corsi, P (2012) Circadian rhythms and memory formation: Regulation by chromatin remodelling. Frontiers in Molecular Neuroscience, 5, p. 37. doi.org/10.3389/fnmol.2012.00037.
- Hsieh, J & Zhao, X (2016) Genetics and Epigenetics in Adult Neurogenesis. Cold Spring Harbor Perspectives in Biology, 8, p. a018911. doi.org/10.1101%2Fcshperspect.a018911.
- Mews, P., et al. (2021) From circuits to chromatin: The emerging role of epigenetics in mental health. Journal of Neuroscience, 41, pp. 873–882. doi.org/10.1523/JNEUROSCI.
- Ma, D. K., et al. (2009) Neuronal Activity–Induced Gadd45b Promotes Epigenetic DNA Demethylation and Adult Neurogenesis. Science, 323, pp. 1074–1077. doi.org/10.1126/science.1166859.
- Yao, B., et al. (2016) Epigenetic mechanisms in neurogenesis. Nature Reviews Neuroscience, 17, pp. 537–549. doi.org/10.1038/nrn.2016.70.
- Vollmayr, B., et al. (2007) Neurogenesis and depression: What animal models tell us about the link. European Archives of Psychiatry and Clinical Neuroscience, 257, pp. 300–303. doi.org/10.1007/s00406-007-0734-2.
- Sharma, R., et al. (2008) Epigenetic targets for melatonin: Induction of histone H3 hyperacetylation and gene expression in C17. 2 neural stem cells. Journal of Pineal Research, 45, pp. 277–284. doi.org/10.1111/j.1600-079X.2008.00587.x.
- Niles, L.P., et al. (2013) Melatonin induces histone hyperacetylation in the rat brain. Neuroscience Letters, 541, pp. 49–53. doi.org/10.1016/j.neulet.2013.01.050.
- Sarkar, A., et al. (2014) Hippocampal HDAC4 contributes to postnatal fluoxetine-evoked depression-like behavior. Neuropsychopharmacology, 39, pp. 2221–2232. doi.org/10.1038/npp.2014.73.
- Schwimmer, H., et al. (2014) Light at night and melatonin have opposite effects on breast cancer tumors in mice assessed by growth rates and global DNA methylation. Chronobiology International, 31, pp. 144–150. doi.org/10.3109/07420528.2013.842925.
- Haim, A & Zubidat, A E (2015) Artificial light at night: Melatonin as a mediator between the environment and epigenome. Proceedings of the Royal Society B: Biological Sciences, 370, p. 20140121. doi.org/10.1098/rstb.2014.0121.
- Rodríguez, A., et al. (2017) Reducing light-induced mortality of seabirds: High pressure sodium lights decrease the fatal attraction of shearwaters. Journal for Nature Conservation, 39, pp. 68–72. doi.org/10.1016/j.jnc.2017.07.001.
- Cabrera-Cruz, S. A., et al. (2018) Light pollution is greatest within migration passage areas for nocturnally-migrating birds around the world. Scientific Reports, 8, p. 3261. doi.org/10.1038/s41598-018-21577-6.
- Loss, S.R., et al. (2014) Bird–building collisions in the United States: Estimates of annual mortality and species vulnerability. The Condor, 116, pp. 8–23. doi.org/10.1650/CONDOR-13-090.1.
- Griffith, S. C., et al. (2021) The Ecology of the Zebra Finch Makes It a Great Laboratory Model but an Outlier amongst Passerine Birds. Birds, 2, pp. 60–76. doi.org/10.3390/birds2010004.
- Fatal Light Awareness Program, Canada. Available online: https://flap.org/.
- Lights Out Program, Audubon Society, USA. Available online: https://www.audubon.org/about .