Decarbonization by 2050 is a primary market objective for countries worldwide, representing a crucial step toward a more sustainable planet and more efficient energy use. It is also a key strategy for addressing the challenges posed by the widespread use of fossil fuels, such as limited fuel supplies, rising fuel prices, and the harmful gas emissions—like SOx, NOx, and CO2—that pollute our atmosphere.
These ongoing issues related to fossil fuel-derived energy have accelerated the search for alternatives, driving the need for greater independence from gas and oil. A complete transition to hydrogen-derived energy is expected to play a pivotal role in the decarbonization of all sectors of the economy.
To achieve this, it is essential to establish a roadmap for renewable hydrogen energy. This involves identifying key priorities and available resources while addressing the challenges associated with the development of renewable hydrogen and potential solutions for overcoming them.
In Spain, efforts are underway to facilitate the deployment of hydrogen energy, with the country’s roadmap aligned with the European Commission’s 2021 Annual Strategy of Sustainable Growth. This work is expected to be central to Spain’s efforts to develop the technology necessary to decarbonize various sectors of its economy.
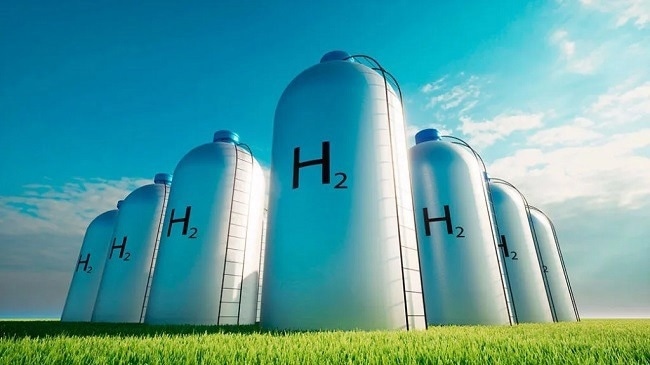
Image Credit: AIMPLAS
What is Hydrogen?
Hydrogen is considered the universe’s most abundant and simplest element, with the potential to provide energy for virtually any type of transport, as well as for commercial, residential, and even industrial buildings.
However, hydrogen is not a primary energy source; it is an energy carrier. Energy must first be produced from a primary (ideally renewable) energy resource, with hydrogen then used to transport and store that energy. When hydrogen is used to carry energy generated from renewable sources, it is referred to as “green hydrogen.”
Data from the International Energy Agency (IEA) highlights that around 70 million tonnes of hydrogen are consumed globally. This hydrogen is primarily used as a reactant in various chemical processes, including ammonia production and the hydrocracking (desulfurization) of fuels. These specific applications account for 80 % of global hydrogen demand.
Once produced, hydrogen’s chemical energy can be converted into different forms through various pathways, a concept known as Power-to-X.
Hydrogen can be transformed into electricity (power-to-power), changed into methane, and/or injected into natural gas (power-to-gas), serving as a primary substance for the chemical industry (power-to-industry). It can also be converted into fuels like methanol (power-to-fuels) or used as a means of mobility (power-to-mobility).
By leveraging these pathways, hydrogen can effectively integrate renewable energies on a large scale across all primary sectors of the economy. It also has the potential to act as a shock absorber, increasing system flexibility and aiding in the decarbonization of energy systems.
The EU has estimated that hydrogen will represent 14 % of its energy mix by 2050. Achieving this goal depends on the maturity of related technologies for widespread deployment. Thus, technological challenges related to cost, production, and the limited distribution capacity of the existing gas network must be addressed.
Advantages of Hydrogen
The use of hydrogen as a means of energy transfer offers numerous advantages, because hydrogen is different to fossil fuels. For example:
- Hydrogen production does not result in CO2 emissions.
- It offers a high density of energy per unit of mass.
- It is extremely volatile.
- Hydrogen requires a low activation energy.
- Hydrogen is non-toxic.
- It possesses a highly inferior limit of flammability and detonation, as well as high spontaneous combustion temperature.
- Hydrogen is safe for use in open spaces.
Types of Hydrogen Production
Three primary approaches to hydrogen production currently exist:
- Non-renewable fossil resources– this includes natural gas, oil, and carbon including uranium.
- Renewable resources– including solar energy, wind, hydraulic, geothermal energy and biomass.
- Nuclear energy– depending on the type of resource and the process that is used to obtain it, hydrogen can be classified in different colours.
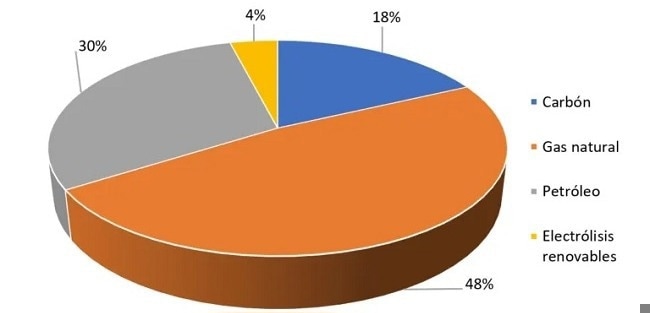
Figure. Hydrogen as an energy vector. Source: Asociación española del hidrógeno, AeH2. Image Credit: AIMPLAS
Hydrogen Production via Non-Renewable Fossil Resources
A total of 95 % of hydrogen is produced using combustibles, with natural gas being the most common. This method of hydrogen production generates significant quantities of CO2, although there are techniques available for recapturing this CO2.
Chemical processes for generating hydrogen based on carbon sources or natural gas include:
- Reforming vapor (grey hydrogen)
- Partial oxidation (grey hydrogen)
- Reforming auto thermal (grey hydrogen)
- Vaporization (blue hydrogen)
- Pyrolysis (turquoise hydrogen)
- Reforming vapor or vaporization by capturing CO2 (blue hydrogen)
Hydrogen Production via Renewable Resources
Just 4 % of hydrogen is currently obtained via renewable resources, although work is ongoing in this area due to hydrogen’s enormous potential as a source of clean energy.
Processes for obtaining renewable hydrogen include:
- Electrolysis: Uses electricity from renewable sources (wind, solar, hydro, biomass) to produce green hydrogen.
- Steam Reforming: Can use renewable energy (wind, solar, hydro, biomass) to produce green hydrogen.
- Biomass Gasification: Utilizes renewable energy (wind, solar, hydro, biomass) to generate green hydrogen.
Hydrogen Production via Nuclear Energy
The production of hydrogen using nuclear energy has the potential to significantly reduce carbon emissions while enhancing profitability in the nuclear sector. Nuclear reactors can be paired with hydrogen production facilities, creating a more efficient system for both energy and hydrogen production through co-generation.
Hydrogen production using nuclear energy can be achieved through:
- Electrolysis (producing purple hydrogen)
- Thermochemical Cycles (producing purple hydrogen)
Hydrogen Storage
Effective hydrogen storage is crucial for the development of a hydrogen-based economy. This represents a significant challenge because hydrogen is difficult to store.
As a gas, hydrogen has a low density of 7 % in air. It condenses to a liquid at -253 °C, with a similar density of 7 % in water. Consequently, storage systems and conditions can vary greatly depending on the hydrogen's intended use.
For generating electric and thermal energy in the residential sector or for decentralized electricity generation in industrial settings, stable storage systems can be utilized. In these cases, there are fewer constraints related to surface area, weight, and volume, as well as less need for peripheral systems.
However, hydrogen storage for automotive applications faces significant constraints related to weight and volume, along with increasingly strict regulations on hydrogen quality set by the EU's Department of Energy. These limitations must be addressed to ensure that hydrogen-powered vehicles can compete with conventional vehicles in terms of performance and availability.
Additional barriers include operating conditions and hydrogen supply kinetics, which have restricted the use of hydrogen in combustible batteries for cars, buses, trucks, and other vehicles. Developing technologies to overcome these barriers—such as improving insulation and creating lighter batteries—is essential.
Furthermore, given hydrogen’s characteristics, safety is a primary consideration when selecting a hydrogen storage system.
A number of options are available, but these storage systems may not be suitable for every application. Example hydrogen storage systems include:
- Gas pressure
- Liquid (cryogenic storage)
- Metal hydrides
- Porous polymers (for example, active carbon, graphite, zeolites, MOF, or COF)
- As chemical compounds (for example, NH3 or toluene)
- In glass microspheres
Of these options, only the first three currently offer sufficient reliability for market presentation and application with certainty. Therefore, there is ample opportunity for further research and exploration.
Projects at AIMPLAS
AIMPLAS is actively addressing the challenges associated with the generation and use of hydrogen as a renewable fuel. The company is involved in several projects across the industrial sector, with the goal of supporting innovation and collaborating with a diverse range of partner companies.
These projects include:
- Evaluation of Alternative Energy Vectors: Assessing energy vectors derived from waste as alternatives to fossil fuels.
- Selective Hydrogen Production: Utilizing catalyst-assisted thermochemical treatment processes for targeted hydrogen production.
- Design and Modification of Materials: Developing and modifying polymeric and nanoporous materials to enhance the effective storage of energy vectors, focusing on improving weight and permeability.
- Viability Study of Hydrogen Generation: Investigating the potential of using complex plastic waste for hydrogen production through valorization techniques.
Acknowledgments
Produced from materials originally authored by Natalia Pons from AIMPLAS.
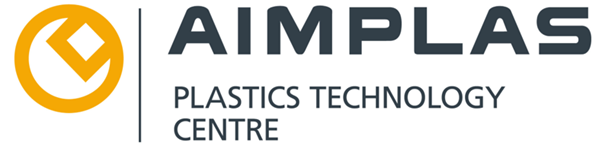
This information has been sourced, reviewed and adapted from materials provided by AIMPLAS.
For more information on this source, please visit AIMPLAS.