Carbon-neutral and carbon-negative technology, such as carbon capture, will be required to reduce CO2 concentrations in the atmosphere to levels that will minimize the consequences of climate change. The energetics of CO2 binding to the proposed capture material must be carefully balanced while developing optimum carbon capture materials. CO2 binding free energies (ΔGbind) are, however, difficult to obtain experimentally.
This article looks at the latest research, titled 'Predictive energetic tuning of C-Nucleophiles for the electrochemical capture of carbon dioxide', published in Iscience.
Among the potential materials for CO2 capture and conversion, imidazolium salts have gotten a lot of interest because of the appealing liquid characteristics of their alkylated forms, which include good CO2 solvation and high conductivity, making them ideal for electrochemical applications.
In addition to these advantageous features, deprotonation of imidazolium salts yields N-heterocyclic carbenes (NHCs) (Scheme 1B). Electrochemical access to the free NHCs has been proven before, as indicated in Scheme 1A.
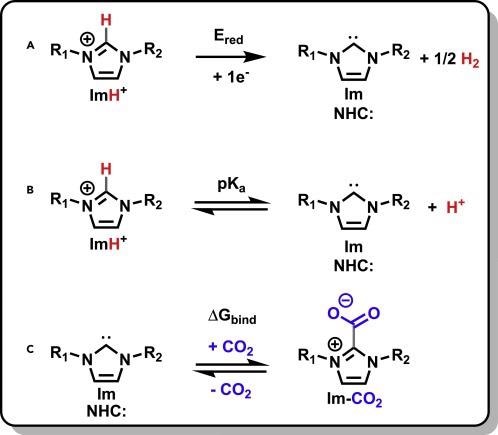
Scheme 1. Reactions of imidazolium cations. Generation of NHCs (Im) from their imidazolium precursors (A and B) and Im binding to CO2 (C) along with the three properties associated with these reactions: (A) Ered, (B) pKa, and (C) ΔGbind. R1 and R2 substituents are described in Figure 1. Scheme Credit: Petersen, et al., 2022
When an imidazolium cation is reduced, it releases half of its aH2 equivalent and becomes a free NHC. As illustrated in Scheme 1C, the free NHC then displays reactivity with CO2. The Scheme 1C reaction was first designed as a protection strategy for reactive NHCs, since it found use in the synthesis of organometallics.
Researchers now show a three-way correlation between the imidazolium’s experimentally accessible reduction potential (Ered) (Scheme 1A), its pKa (Scheme 1B), and the CO2 binding energy of the associated NHC using this reactivity (Scheme 1C). On a set of 90 imidazolium, the correlation between the pKa of an imidazolium (Scheme 1B) and the ΔGbind of the corresponding free NHCs (Scheme 1C) was investigated using density functional theory (DFT).
Researchers depict a landscape of CO2 binding free energies spanning 15 kcal, 20 pKa units, and 1.7 V of potential in this work, even though the number of imidazolium salts is smaller (Figure 1).
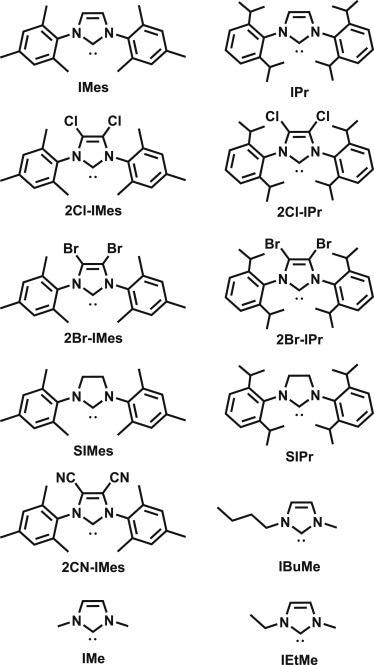
Figure 1. Imidazol(in)ium-based NHCs used in the correlation studies. Structures of each compound under study. See also Figure S1 and Table S1 for applicable experimental values associated with each NHC. Imidazolium counterparts (protonated NHCs) are abbreviated as “NHC”H in this work. Image Credit: Petersen, et al., 2022
In Scheme 1, scientists study the relation between Ered, pKa, and ΔGbind for reactions A–C. Due to their relative dependency on the electron density at the apical imidazolium carbon, the parameters are linked.
In essence, the nucleophilicity of an NHC is ranked against two types of electrophiles: protons and carbon dioxide, according to the association of pKa with the free energy of CO2 binding.
Unlike conventional electro-swing systems, which cycle through binding CO2 with cathodically generated nucleophiles and then release CO2 in an electrochemical oxidation step, the electrochemical step of NHC generation and binding CO2 can be combined with a thermal release step rather than oxidation. Because the thermal emission of CO2 from NHC adducts is known, hybrid electrothermal CO2 capture-release systems may be developed.
Methodology
A silver wire single-junction pseudo-reference electrode, a 3 mm diameter glassy carbon working electrode (MF-2012, BASI), and a platinum wire counter electrode were used in normal pulse voltammetry (NPV) studies. This determined the potential at which the inflection point in the NPV of the molecule in question yielded experimental imidazolium reduction potentials.
Because of the irreversible nature of the lowering and the enhanced capacity of NPV to deliver exact current responses with low interference from charging currents, this approach was chosen over the conventional use of cyclic voltammetry E1/2 values. After that, the value was averaged over three trials (except SIPr, 6 trials).
Diffusion-ordered spectroscopy (DOSY) NMR on a sample of 1,3-bis(2,6-diisopropylphenyl)-4,5-dihydroimidazolium tetrafluoroborate (SIPrH BF4) in deuterated MeCN was used to determine the diffusion coefficient of SIPrH.
The optimal geometries and energy of the carbene precursor, its protonated and reductive intermediates, and their adducts with CO2 were computed using quantum chemical density functional theory simulations.
The SMD-Bondi cavity treatment greatly increases the chances of binding. Researchers decided that the default SMD treatment with the MN15 functional delivers more accurate binding free energy values and chose to employ this technique for the correlations since it is the least tested across multiple systems and hence the least trustworthy.
The linear fit between experimentally measured pKa values and the estimated free energy difference G(XH+) – G(X) yields pKa values. Whereas, it is normal to utilize an isodesmic technique or pre-determined free energy of solvated protons G(H+) to estimate pKa values.
In OriginPro 2019b, best-fit studies of the associated parameters (Ered vs. pKa, Ered vs. ΔGbind, pKa vs. ΔGbind, and Ered vs. pKa vs. ΔGbind) were performed.
Results
Scientists initially aimed to experimentally test many assumptions about the system before undertaking DFT calculations to study the link with the free energy of CO2 binding, reduction potential, and pKa for the set of imidazolium cations. Aromatic hydrides have previously been demonstrated to have a linear relationship between the oxidized hydride’s first reduction potential and the hydride’s nucleophilicity.
Researchers next used DFT based on the MN15/6-311+G(d,p) level of theory combined with the SMD solvent model as performed in the Gaussian 16 quantum chemistry code to calculate the values of Ered, pKa, and ΔGbind for each of the imidazolium derivatives.
Figure 2 shows imidazolium cations that are difficult to make synthetically. Correlations were computed using the estimated DFT values for Ered and pKa in these circumstances.
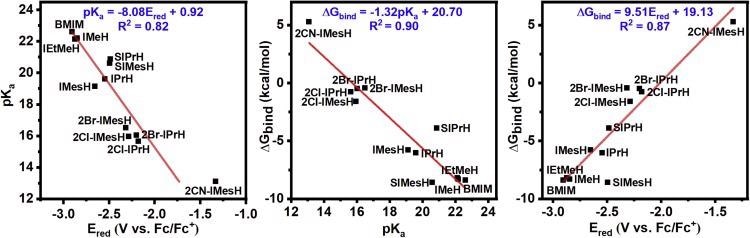
Figure 2. Linear correlations of pKa, free energy of CO2 binding, and reduction potential. The three linear two-way correlations of (left) reduction potential and pKa, (middle) free energy of CO2 binding and pKa, and (right) reduction potential and free energy of CO2 binding for the compounds under study. Optimized molecular coordinates are available in Data S1. Image Credit: Petersen, et al., 2022
In the case of imidazol(in)ium cations, where both the experimental pKa and Ered are known in advance depicts in Figure 3.
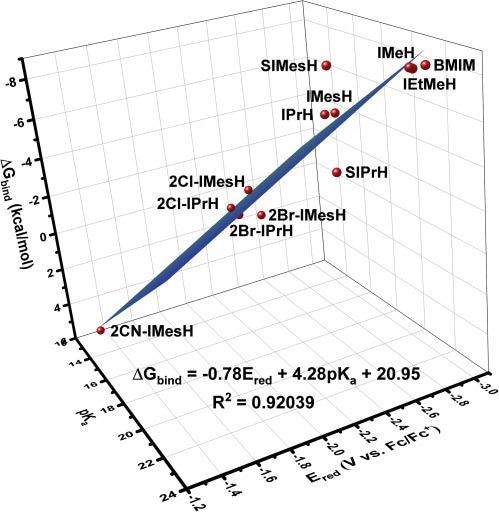
Figure 3. 3D correlation provides greater predictive power for estimating the free energy of CO2 binding. Three-parameter linear relationship providing stronger correlation between Ered, pKa, and ΔGbind for the compounds under study. Image Credit: Petersen, et al., 2022
Table 1 shows the results for all six substances for which experimental Ered and pKa values were obtained.
Table 1. Comparison of DFT-calculated ΔGbind values with those estimated using experimental reduction potentials and pKa value. Source: Petersen, et al., 2022
ImH |
Exp. Ered (V vs. Fc/Fc+) |
Exp. pKa |
Est. ΔGbind (kcal/mol) |
DFT ΔGbind (kcal/mol) |
Error (%) |
IMesH |
–2.64 |
19.4 |
–5.4 |
–5.8 |
6 |
IPrH |
–2.63 |
19.3 |
–5.3 |
–6.0 |
12 |
IMeH |
–2.83 |
22.0 |
–8.3 |
–8.3 |
0.2 |
EtMeH |
–2.87 |
22.1 |
–8.5 |
–8.2 |
4 |
BMIM |
–2.84 |
22.0 |
–8.3 |
–8.4 |
1 |
The calculated and experimental pKas are compared in Table 2.
Table 2. Comparison of estimated pKa values from Equation 4 and experimental pKa values. Source: Petersen, et al., 2022
ImH |
Exp. Ered (V vs. Fc/Fc+) |
Est. pKa |
Exp. pKa |
Error (%) |
IMesH |
–2.64 |
22.2 |
19.4 |
15 |
IPrH |
–2.63 |
22.2 |
19.3 |
15 |
IMeH |
–2.83 |
23.8 |
22.0 |
8 |
EtMeH |
–2.87 |
24.1 |
22.1 |
9 |
BMIM |
–2.84 |
23.9 |
22.0 |
8 |
Discussion
Researchers now show how to calculate CO2 binding energies from experimentally accessible electrochemical data by establishing a three-way connection between pKa, reduction potential, and free energy of CO2 binding for a group of imidazol(in)ium cations and the corresponding NHCs.
Four halogenated NHCs that are reported to be oxygen stable, an essential sorbent property for carbon capture applications involving oxygen, were expected to bind CO2 with negative ΔGbind values.
The strong ionic connection may affect measured reduction potentials, yielding values that are significantly different from those anticipated by the correlations provided here. Researchers anticipate the association to remain over a vast library of chemically related species, even though the subset of organic salts studied was constrained. However, as shown in the case of 1,3-di-t-butylimidazolium chloride (ItBuHCl), steric effects can cause significant variations from recognized patterns.
Conclusion
While researchers show these predictions for imidazol(in)ium-based NHCs in this work, identical correlations will apply to other groups of electrochemically responsive CO2 capture molecular materials, allowing for the mapping of the energetics of novel chemical space for this application.
These findings, when combined with the previously discovered thermally induced CO2 release from NHC-CO2 adducts, pave the way for energetic modulation of reactive separations in new hybrid redox-thermal swing chemistries.
Journal Reference
Petersen, H.A., Alherz, A.W., Stinson, T.A., Huntzinger, C.G., Musgrave, C.B. and Luca, O.R. (2022) Predictive energetic tuning of C-Nucleophiles for the electrochemical capture of carbon dioxide. Iscience, p.103997. Available Online: https://www.cell.com/iscience/fulltext/S2589-0042(22)00267X?_returnURL=https%3A%2F%2Flinkinghub.elsevier.com%2Fretrieve%2Fpii%2FS258900422200267X%3Fshowall%3Dtrue.
References and Further Reading
- Alherz, A., et al. (2018) Renewable hydride donors for the catalytic reduction of CO2: a thermodynamic and kinetic study. The Journal of Physical Chemistry B, 122, pp. 10179–10189. doi.org/10.1021/acs.jpcb.8b08536.
- Arduengo A.J., et al. (1997) An air stable carbene and mixed carbene “dimers”. Journal of American Chemical Society, 119, pp. 12742–12749. doi.org/10.1021/ja973241o.
- Barone V & Cossi M (1998) Quantum calculation of molecular energies and energy gradients in solution by a conductor solvent model. Journal of Physical Chemistry A, 102, pp. 1995–2001. doi.org/10.1021/jp9716997.
- Camaioni D M & Schwerdtfeger C A (2005) Comment on “accurate experimental values for the free energies of hydration of H+, OH-, and H3O+”. Journal of Physical Chemistry A, 109, pp. 10795–10797. doi.org/10.1021/jp054088k.
- Chai J.-D & Head-Gordon M (2008) Long-range corrected hybrid density functionals with damped atom–atom dispersion corrections. Physical Chemistry Chemical Physics, 10, p. 6615. doi.org/10.1039/B810189B.
- Chu Y., et al. (2007) An acidity scale of 1,3-dialkylimidazolium salts in dimethyl sulfoxide solution. The Journal of Organic Chemistry, 72, pp. 7790–7793. doi.org/10.1021/jo070973i.
- Cossi M., et al. (2003) Energies, structures, and electronic properties of molecules in solution with the C-PCM solvation model. Journal of Computational Chemistry, 24, pp. 669–681. doi.org/10.1002/jcc.10189.
- Donadt T. B., et al. (2018) DOSY NMR and normal pulse voltammetry for the expeditious determination of number of electrons exchanged in redox events. ChemistrySelect, 3, pp. 7410–7415. doi.org/10.1002/slct.201801231.
- Dunn M.H., et al. (2017) Targeted and systematic approach to the study of pKa values of imidazolium salts in dimethyl sulfoxide. Journal of Computational Chemistry, 82, pp. 7324–7331. doi.org/10.1021/acs.joc.7b00716.
- Frisch M. J., et al. (2016) Gaussian 16 Rev. C.01. Available at https://gaussian.com/citation.
- Furfari S.K., et al. (2015) Air stable NHCs: a study of stereoelectronics and metallorganic catalytic activity. Chemical Communications, 51, pp. 74–76. doi.org/10.1039/C4CC06809B.
- Gorodetsky B., et al. (2004) Electrochemical reduction of an imidazolium cation: a convenient preparation of imidazol-2-ylidenes and their observation in an ionic liquid. Chemical Communications, 2004, pp. 1972–1973. https://doi.org/10.1039/B407386J.
- Hahn F E & Jahnke M C (2008) Heterocyclic carbenes: synthesis and coordination chemistry. Angewandte Chemical International Edition, 47, pp. 3122–3172. doi.org/10.1002/anie.200703883
- Ilic S., et al. (2018) Thermodynamic and kinetic hydricities of metal-free hydrides. Chemical Society Reviews, 47, pp. 2809–2836. doi.org/10.1039/C7CS00171A
- Institute G.C (2020) The Global Status of CCS: 2020 (Australia)
- Isse A A & Gennaro A (2010) Absolute potential of the standard hydrogen electrode and the problem of interconversion of potentials in different solvents. Journal of Physical Chemistry B, 114, pp. 7894–7899. doi.org/10.1021/jp100402x.
- Kelemen Z., et al. (2011) An organocatalytic ionic liquid. Organic and Biomolecular Chemistry, 9, pp. 5362–5364. doi.org/10.1039/C1OB05639E
- Kelly C.P., et al. (2006) Aqueous solvation free energies of ions and ion−water clusters based on an accurate value for the absolute aqueous solvation free energy of the proton. Journal of Physical Chemistry B, 110, pp. 16066–16081. doi.org/10.1021/jp063552y
- Krishnan R., et al. (1980) Self-consistent molecular orbital methods. XX. A basis set for correlated wave functions. The Journal of Chemical Physics, 72, pp. 650–654. doi.org/10.1063/1.438955
- Lackner K S (2013) The thermodynamics of direct air capture of carbon dioxide. Energy, 50, pp. 38–46. doi.org/10.1016/j.energy.2012.09.012.
- Luca O R & Fenwick A Q (2015) Organic reactions for the electrochemical and photochemical production of chemical fuels from CO2–The reduction chemistry of carboxylic acids and derivatives as bent CO2 surrogates. Journal of Photochemistry and Photobiology B: Biology, 152, pp. 26–42. doi.org/10.1016/j.jphotobiol.2015.04.015
- Luca O. R., et al. (2015) The selective electrochemical conversion of preactivated CO2 to methane. Journal of Electrochemical Society, 162, p. H473. doi.org/10.1149/2.0371507jes
- Marenich A.V., et al. (2009) Universal solvation model based on solute electron density and on a continuum model of the solvent defined by the bulk dielectric constant and atomic surface tensions. Journal of Physical Chemistry B, 113: 6378–6396. doi.org/10.1021/jp810292n
- Marenich A.V., et al. (2014) Computational electrochemistry: prediction of liquid-phase reduction potentials. Physical Chemistry Chemical Physics, 16, pp. 15068–15106. doi.org/10.1039/C4CP01572J
- McLean A D & Chandler G S (1980) Contracted gaussian basis sets for molecular calculations. I. second row atoms, Z=11–18. The Journal of Chemical Physics, 72, pp. 5639–5648. doi.org/10.1063/1.438980.
- Mirzaei S., et al. (2019) Improving performance of the SMD solvation model: bondi radii improve predicted aqueous solvation free energies of ions and pKa values of thiols. Journal of Physical Chemistry A, 123, pp. 9498–9504. doi.org/10.1021/acs.jpca.9b02340
- Namazian M., et al. (2010) Benchmark calculations of absolute reduction potential of ferricinium/ferrocene couple in nonaqueous solutions. Journal of Chemical Theory and Computation, 6, pp. 2721–2725. doi.org/10.1021/ct1003252
- (2019) National Academies of Sciences, Engineering, and Medicine Negative Emissions Technologies and Reliable Sequestration: A Research Agenda. The National Academies Press.
- Petersen H A & Luca O R (2021) Application-specific thermodynamic favorability zones for direct air capture of carbon dioxide. Physical Chemistry Chemical Physics, 23, pp. 12533–12536. doi.org/10.1039/D1CP01670A
- Riduan S.N., et al. (2009) Conversion of carbon dioxide into methanol with silanes over N-heterocyclic carbene catalysts. Angewandte Chemie International Edition, 48, pp. 3322–3325. doi.org/10.1002/anie.200806058
- Sowmiah S., et al. (2009) On the chemical stabilities of ionic liquids. Molecules, 14, pp. 3780–3813. doi.org/10.3390/molecules14093780
- Thapa B & Schlegel H B (2016) Density functional theory calculation of pKa’s of thiols in aqueous solution using explicit water molecules and the polarizable continuum model. Journal of Physical Chemistry A, 120, pp. 5726–5735. doi.org/10.1021/acs.jpca.6b05040
- Tossell J A (2011) Calculation of the properties of molecules in the pyridine catalyst system for the photochemical conversion of CO2 to methanol. Computational and Theoretical Chemistry, 977, pp, 123–127. doi.org/10.1016/j.comptc.2011.09.012
- Tsuzuki S., et al. (2013) Interactions in ion pairs of protic ionic liquids: comparison with aprotic ionic liquids. Journal of Chemical Physics, 139, p. 174504. doi.org/10.1063/1.4827519
- Van Ausdall B.R., et al. (2009) A systematic investigation of factors influencing the decarboxylation of imidazolium carboxylates. Journal of Organic Chemistry, 74, pp. pp. 7935–7942. doi.org/10.1021/jo901791k.
- Voroshylova I.V., et al. (2018) Influence of the anion on the properties of ionic liquid mixtures: a molecular dynamics study. Physical Chemistry Chemical Physics, 20, pp. 14899–14918. doi.org/10.1039/C8CP01541D
- Voutchkova A.M., et al. (2007) Imidazolium carboxylates as versatile and selective N-heterocyclic carbene transfer agents: synthesis, mechanism, and applications. Journal of American Chemical Society, 129, pp. 12834–12846. doi.org/10.1021/ja0742885
- Wang H., et al. (2015) Anion-effects on electrochemical properties of ionic liquid electrolytes for rechargeable aluminum batteries. Journal of Materials Chemistry A, 3, pp. 22677–22686. doi.org/10.1039/C5TA06187C
- Wang Z., et al. (2018) Acidity scale of N-heterocyclic carbene precursors: can we predict the stability of NHC–CO2 adducts?. Organic Letters, 20, pp. 6041–6045. doi.org/10.1021/acs.orglett.8b02290
- Wilcox J (2020) An electro-swing approach. Nature Energy, 5, pp. 121–122. doi.org/10.1038/s41560-020-0554-4.
- Yang L & Wang H (2016) MN15: a kohn–sham global-hybrid exchange–correlation density functional with broad accuracy for multi-reference and single-reference systems and noncovalent interactions. Chemical Science, 7, pp. 962–998. doi.org/10.1039/C6SC00705H
- Yu H.S., et al. (2016) MN15: a kohn–sham global-hybrid exchange–correlation density functional with broad accuracy for multi-reference and single-reference systems and noncovalent interactions. Chemical Science, 7, pp. 5032–5051. doi.org/10.1039/C6SC00705H
- Zhao S.-F., et al. (2016) Is the imidazolium cation a unique promoter for electrocatalytic reduction of carbon dioxide?. The Journal of Physical Chemistry C, 120, pp. 23989–24001. doi.org/10.1021/acs.jpcc.6b08182