Ecosystems in various parts of the world are impacted by global environmental change. Forests are important terrestrial ecosystems where evidence is mounting of cascading effects from anthropogenic-induced climate change, with far implications for the water and carbon (C) cycles, as well as societal services.
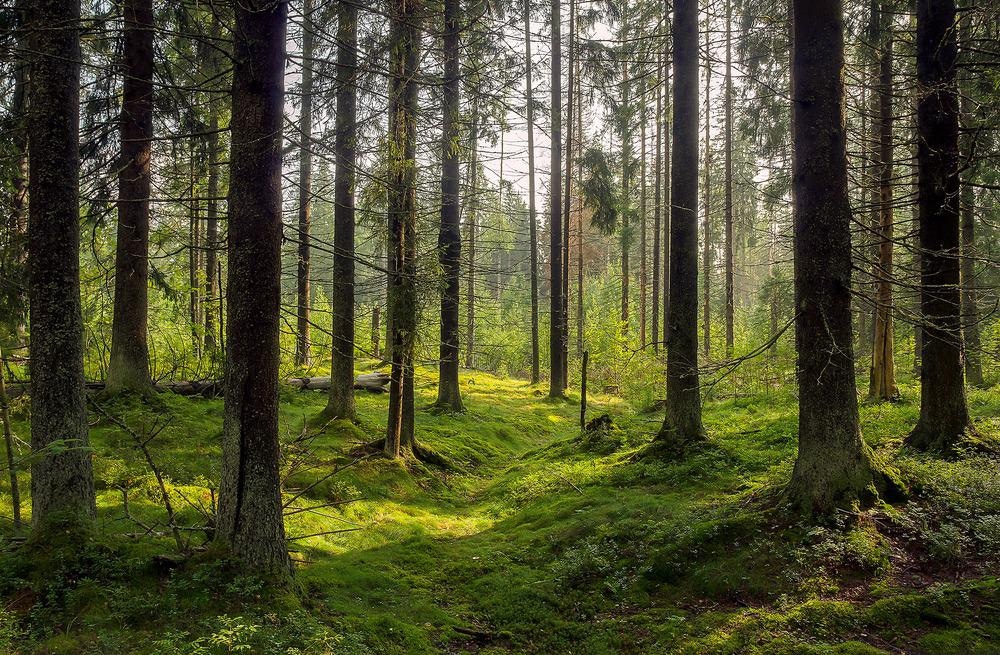
Image Credit: Lillac/Shutterstock.com
Large-scale assessments including the whole distribution range of major species can be used to answer evolving questions about those consequences.
This article looks at recent research published in Communications Biology, which looks at the climate-change-driven growth decline of European beech forests.
Forest cover prediction research has a long history of focusing on the relationship between climate change and forest presence/abundance. Ecologically based projections of species growth performance are less well understood.
Local data is often presented in dendroecological analyses, which have provided a useful regional understanding of growth responses to local habitat conditions and climate change. Despite current breakthroughs in tree-ring research, spatio-temporal investigations of actual and expected growth, particularly at scales that include species’ geographic and climatic distributions, are rare.
International dendrochronological databanks have been produced by the tree-ring community, however, they are often biased or limited in species, biomes, and trailing-edge populations, limiting their utility for ecologically focused applications.
However, extensive networks encompassing the whole variety of bioclimatic and ecological conditions of the subject species are required for the successful upscaling of tree-ring data.
To make this possible, researchers built a rich and species-specific network that supports comparative ecological analysis, with over 780,000 ring width measurements from 5800 trees and 324 sample sites across Europe, covering the complete biological spectrum of Fagus sylvatica L. (hereinafter beech) (Figure 1).
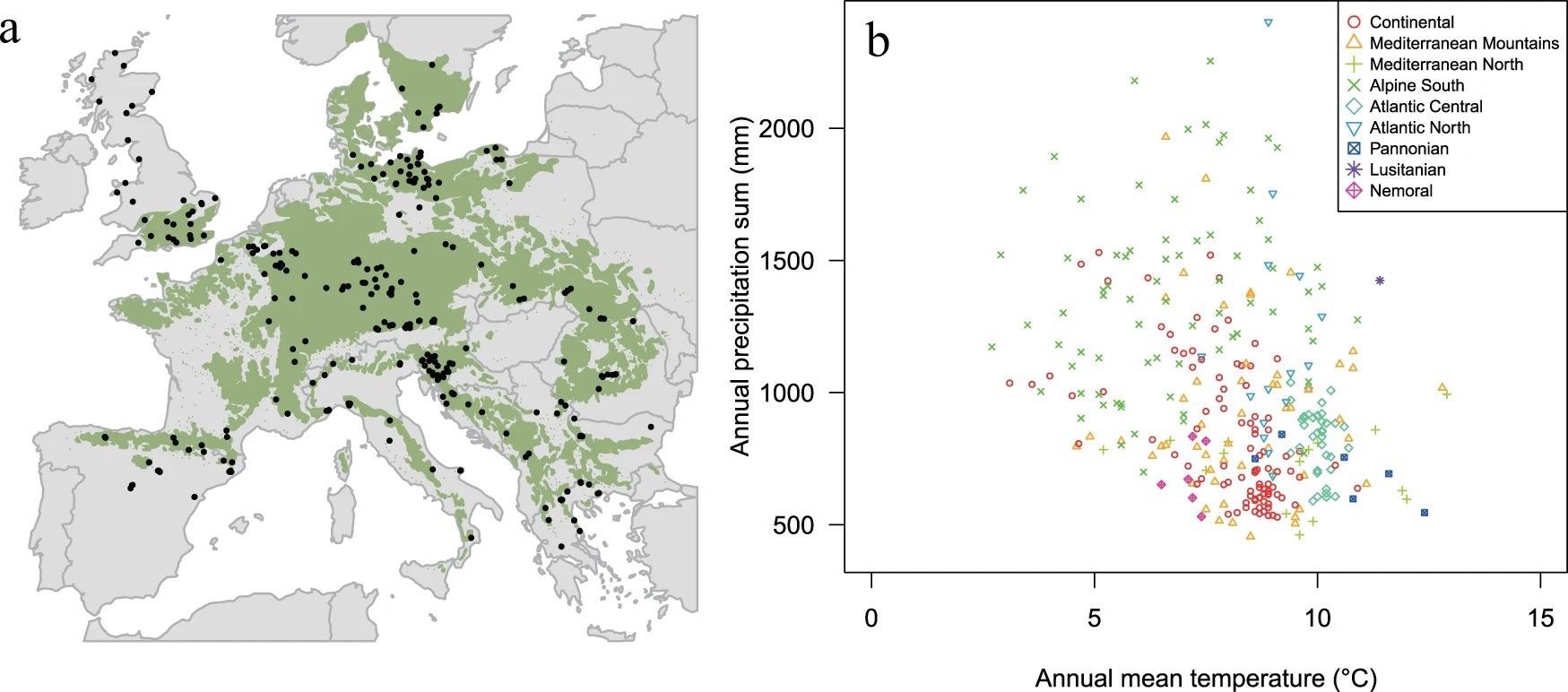
Figure 1. Spatial and climatic range of beech sites. A. Geographical distribution of the 324 study sites (black dots) in the natural distribution range of European beech (green area based on the EUFORGEN map. B. Climatic envelope of European beech sampling sites, considering annual temperature and precipitation. Sites are labeled according to the environmental zones detailed in Metzger et al. Image Credit: Caudullo, et al., 2017; Metzger, et al., 2005
From an ecological and socioeconomic standpoint, beech is among Europe’s most important forest species. Beech played a significant part in postglacial reforestation, spreading fast from its Mediterranean refuges to the continent’s central and northern areas. Beech is presently endangered in its geographical and ecological range due to fast climate change.
The findings show that this species’ development patterns changed dramatically over time, particularly in the southern regions. The simulations revealed that recent expansion has been constrained by climate and modified by site-specific variables.
In this regard, predicted decreases in precipitation and temperature increases would result in a fall in total tree productivity, especially if both occur at the same time. Even in the worst-case climate change situation, tree growth could be greatly accelerated at high latitudes in the future.
Results and Discussion
According to the Akaike Information Criterion (AIC) values, the entire model encompassing all examined and significant variables and interactions was the most accurate in predicting the species’ development across Europe (Table 1).
Table 1. Models’ validation. Each row represents a single model and each colored column represents the inclusion of each group of predictor variables (red, moisture conditions (AI), blue, seasonal climatic temperature and precipitation variables (Cli), yellow, geographical variables (Geo), and green, random effects (RE)). For each model, Akaike Information Criterion value and difference, χ2 test value and degrees of freedom (Df) of the χ2 test and p (Chisq, Pr > Chisq)) are shown. Source: Martinez del Castillo, et al., 2022
|
AI |
Cli |
Geo |
RE |
AIC |
ΔAIC |
Chisq |
Df |
Pr |
Null model |
– |
– |
– |
● |
642591.5 |
25404.4 |
NA |
NA |
NA |
● |
– |
– |
● |
642509.6 |
25322.5 |
83.9 |
1 |
5.32E-20 |
– |
– |
● |
● |
642417.9 |
25230.8 |
95.8 |
2 |
1.61E-21 |
● |
– |
● |
● |
642348.3 |
25161.2 |
77.6 |
4 |
5.66E-16 |
– |
● |
– |
● |
624421.0 |
7233.9 |
17949 |
11 |
0 |
● |
● |
– |
● |
621819.4 |
4632.3 |
2639.6 |
19 |
0 |
– |
● |
● |
● |
618820.9 |
1633.9 |
3038.4 |
20 |
0 |
Full model |
● |
● |
● |
● |
617187.1 |
0.0 |
1647.9 |
7 |
0 |
The GLMM was used to show that the interaction of geographical variables like latitude and altitude with the aridity index (AI) was important in explaining beech growth variability.
Variable interactions have a significant impact on model beech development. Regardless of the relative importance and weight of each seasonal climatic variable, the findings reveal that mean aridity, as well as the geographic components altitude and latitude, regulate the majority of sensitivity to a yearly shifting climate.
Mean growth rates of two successive 31-year periods were calculated for a population-wide typical beech tree with a stable basal area of 86059.03 to evaluate beech forest performance in the past decades (Figure 2).
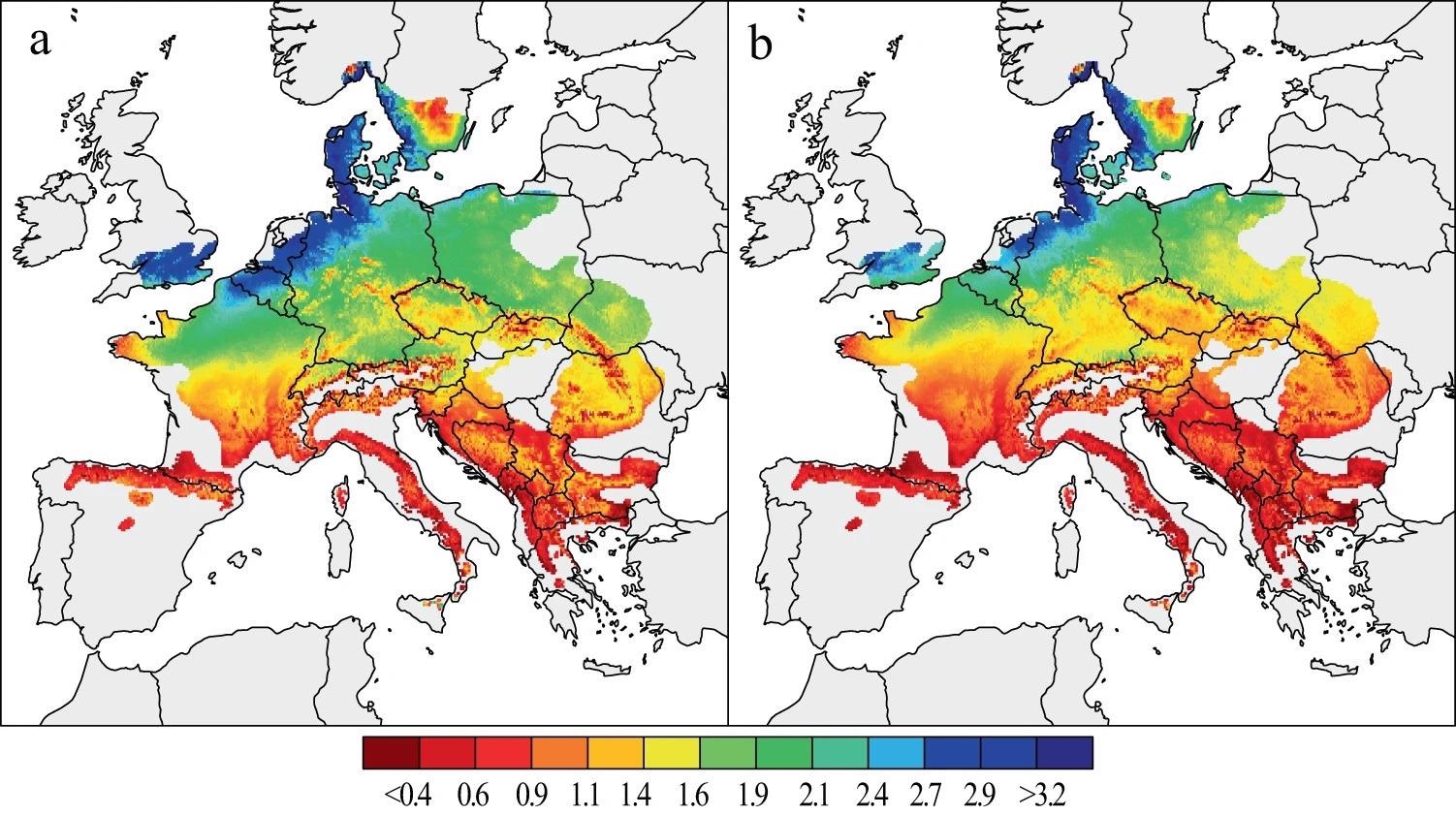
Figure 2. Spatial patterns of beech growth during the last decades. Mean estimates of BAI (in mm2) from 1955–1985 (a) and 1986–2016 (b), were calculated for a theoretical tree derived from a 324-site chronology network. Image Credit: Martinez del Castillo, et al., 2022
The findings demonstrate significant spatial and temporal disparities in beech development across Europe over the last six decades (Figure 3). When compared to the southern distribution limit of beech, the tree growth rate was substantially greater at low altitudes in NW and central Europe, especially coastal sites in Belgium, the Netherlands, Denmark, and the British Isles. At higher altitudes in central Europe, the Alps, and along the Carpathians, lower tree growth is also modeled.
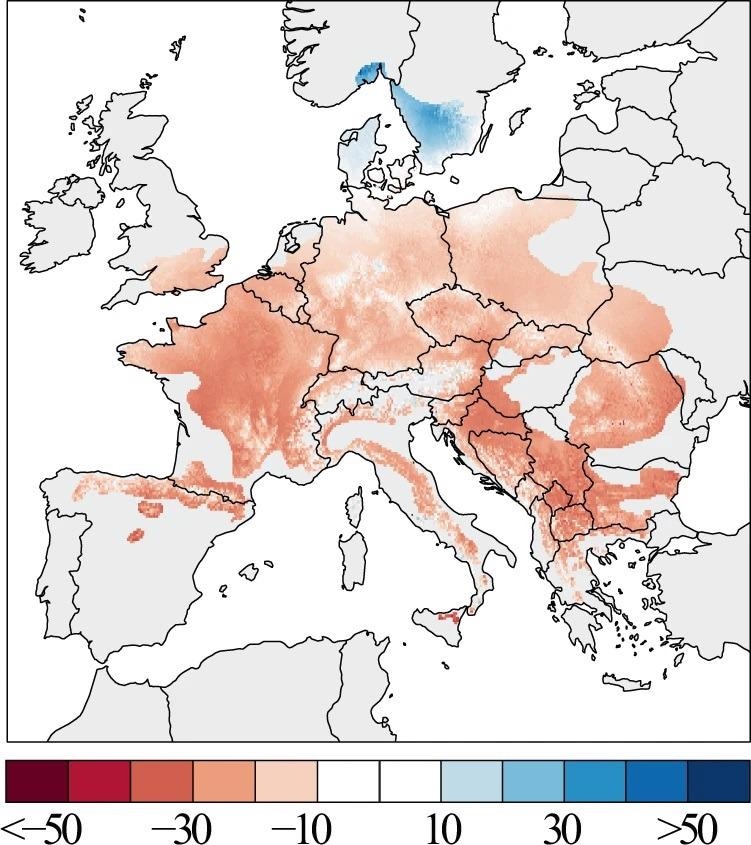
Figure 3. The spatial pattern of beech growth changes across Europe. Tree growth changes are expressed in percent BAI change from 1986 to 2016 relative to the 1955–1985 period mean. Image Credit: Martinez del Castillo, et al., 2022
The spatial representation of growth variations from 1955 to 1985 and from 1986 to 2016 demonstrates a significant drop in growth across the majority of the European beech range (Figure 3). The strength of this reduction varies across Europe, with lesser strength at low latitudes and increasing strength at higher latitudes, suggesting a distinctive latitudinal gradient of forest growth decline.
Under various climate change scenarios, the GLMM produces variable BAI patterns (Figure 4). Even in the more optimistic SSP1-2.6 scenario, growth differences across geographic gradients are larger than the observed growth changes between the two historical periods. Southern Europe’s growth is expected to slow by up to 30% between 2020 and 2050, relative to a 1986–2016 baseline (Figure 4a).
Apart from more pronounced growth declines in southern Europe, such as the Balkans, and more polarized patterns towards the end of the 21st century, the patterns from 2040–2070 and 2060–2090 (Figure 4b, c) are comparable.
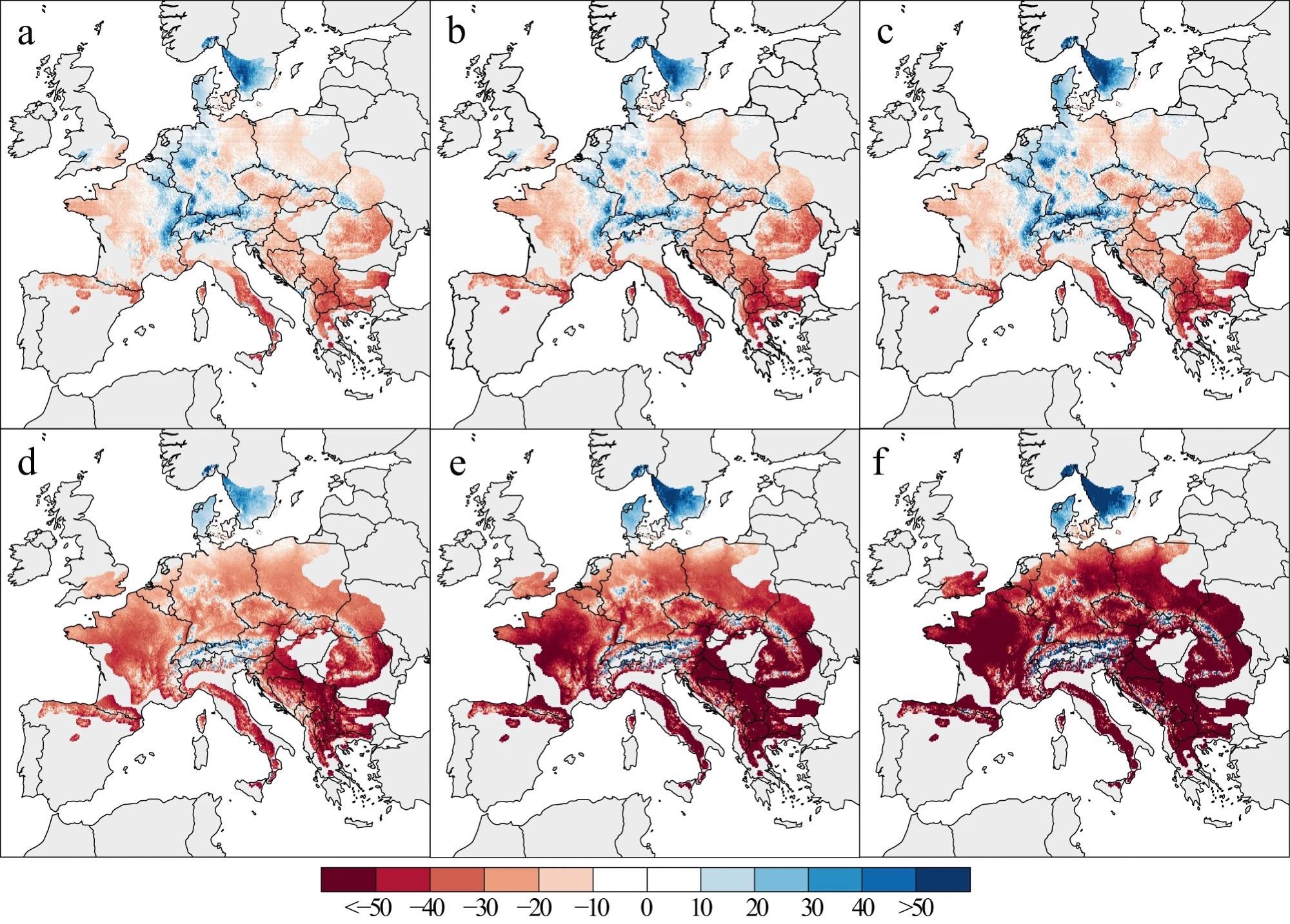
Figure 4. Relative changes in tree growth. Changes are projected under SSP1-2.6 (a–c) and SSP5-8.5 (d–f) CMIP6 climate scenarios for different periods: 2020–2050 (a, d), 2040–2070 (b, e), and 2060–2090 (c, f) In this panel, BAI changes were expressed in percentage of change compared to the 1986–2016 period. Image Credit: Martinez del Castillo, et al., 2022
Figure 4d–f shows how the more likely SSP5-8.5 scenario results in substantial declines in beech output throughout wide swaths of Europe.
The research reveals dramatic changes in the growth patterns of a significant European tree species during the last 60 years and the next 80 years. Growth decreases have been particularly severe in recent decades towards Europe’s southern distribution boundaries, and these general patterns will continue as the climate warms and becomes drier.
GLMM models show that variations in temperature and water availability, which are all controlled by site-specific climate variables, account for the majority of growth disparities.
Moreover, the findings corroborate recent claims of growth slowdowns in Central Europe. In this way, this research bridges gaps between studies and offers a comprehensive analysis, indicating a steady decline in beech tree productivity and carbon sequestration during the 1980s in the most northern parts of the species’ range.
Adaptive management is critical for ensuring forest viability and limiting climate change’s negative consequences. Researchers must determine species-specific climate effects at various spatiotemporal scales to implement such management measures. In this way, empirical modeling has aided forest managers in anticipating climate influences on future forest growth, allowing for species selection in tree plantations or helping migration planning, for example.
Conclusion
Researchers find a prevalent growth rate drop from 1955 to 2016 after analyzing the causes of growth across an unprecedented network of beech sites across Europe. This reduction is widespread across Europe, except in northern distribution range locations in Denmark, Norway, and Sweden, as well as higher elevations in mountainous areas.
Since falling growth has been observed as a prelude to tree die-off, these major growth changes suggest greater forest mortality. These findings cast doubt on recent projections of rising terrestrial carbon stocks because of climate change, given the strength of beech forests as a carbon sink is expected to diminish.
Journal Reference
Martinez del Castillo, E., Zang, C. S., Buras, A., Hacket-Pain, A., Esper, J., Serrano-Notivoli, R., Hartl, C., Weigel, R., Klesse, S., Resco de Dios, V., Scharnweber, T., Dorado-Liñán, I., van der Maaten-Theunissen, M., van der Maaten, E., Jump, A., Mikac, S., Banzragch, B-E., Beck, W., Cavin, L., Claessens, H., Čada, V., Čufar, K., Dulamsuren, C., Gričar, J., Gil-Pelegrín, E., Janda, P., Kazimirovic, M., Kreyling, J., Latte, N., Leuschner, C., Longares, L. A., Menzel, A., Merela, M., Motta, R., Muffler, L., Nola, P., Petritan, A. M., Petritan, I. C., Prislan, P., Rubio-Cuadrado, Á., Rydval, M., Stajić, B., Svoboda, M., Toromani, E., Trotsiuk, V., Wilmking, M., Zlatanov, T., de Luis, M. (2022) Climate-change-driven growth decline of European beech forests. Communications Biology, 5(1), p. 1-9. Available Online: https://www.nature.com/articles/s42003-022-03107-3#citeas.
References and Further Reading
- IPCC. (2014) IPCC Fifth Assessment Report (AR5). 10–12.
- Cailleret, M., et al. (2017) A synthesis of radial growth patterns preceding tree mortality. Global Change Biology, 23, pp. 1675–1690. doi.org/10.1111/gcb.13535.
- Forzieri, G., et al. (2021) Emergent vulnerability to climate-driven disturbances in European forests. Nature Communications, 12, pp. 1–12. doi.org/10.1038/s41467-021-21399-7.
- Bonan, G. B. (2008) Forests and climate change: forcings, feedbacks, and the climate benefits of forests. Science. doi.org/10.1126/science.1155121.
- Buras, A. & Menzel, A. (2019) Projecting tree species composition changes of European forests for 2061–2090 under RCP 4.5 and RCP 8.5 scenarios. Frontiers in Plant Science, 9, pp. 1–13. doi.org/10.3389/fpls.2018.01986.
- van der Maaten, E., et al. (2017) Species distribution models predict temporal but not spatial variation in forest growth. Ecology and Evolution, 7, pp. 2585–2594. doi.org/10.1002/ece3.2696.
- Lebaube, S., et al. (2000) Carbon balance and tree growth in a Fagus sylvatica stand. Annals of Forest Science, 57, pp. 49–61. doi.org/10.1051/forest:2000100.
- Dobbertin, M. (2005) Tree growth as indicator of tree vitality and of tree reaction to environmental stress: a review. European Journal of Forest Research, 124, pp. 319–333. doi.org/10.1007/s10342-005-0085-3.
- Büntgen, U. (2019) Re-thinking the boundaries of dendrochronology. Dendrochronologia, 53, pp. 1–4.
- Klesse, S., et al. (2020) Continental-scale tree-ring-based projection of Douglas-fir growth: Testing the limits of space-for-time substitution. Global Change Biology, 26, pp. 5146–5163. doi.org/10.1111/gcb.15170.
- Zhao, S., et al. (2019) The International Tree-Ring Data Bank (ITRDB) revisited: data availability and global ecological representativity. Journal of Biogeography, 46, pp. 355–368. doi.org/10.1111/jbi.13488.
- Babst, F., et al. (2018) When tree rings go global: challenges and opportunities for retro- and prospective insight. Quaternary Science Reviews, 197, pp. 1–20. doi.org/10.1016/j.quascirev.2018.07.009.
- Klesse, S., et al. (2018) Sampling bias overestimates climate change impacts on forest growth in the southwestern United States. Nature Communications, 9, pp. 1–9. doi.org/10.1038/s41467-018-07800-y.
- Yousefpour, R. et al. (2018) Realizing mitigation efficiency of European commercial forests by climate smart forestry. Scientific Reports, 8, pp. 1–11. doi.org/10.1038/s41598-017-18778-w.
- Giesecke, T., et al. (2007). Towards an understanding of the Holocene distribution of Fagus sylvatica L. Journal of Biogeography, 34, pp. 118–131. doi.org/10.1111/j.1365-2699.2006.01580.x.
- Fang, J. & Lechowicz, M. J. (2006) Climatic limits for the present distribution of beech (Fagus L.) species in the world. Journal of Biogeography, 33, pp. 1804–1819. doi.org/10.1111/j.1365-2699.2006.01533.x.
- Luterbacher, J., et al. (2004) European seasonal and annual temperature variability, trends, and extremes since 1500. Science, 303, pp. 1499–1503. doi.org/10.1126/science.1093877.
- Luterbacher, J., et al. (2016) European summer temperatures since Roman times. Environmental Research Letters, 11, p. 24001. doi.org/10.1088/1748-9326/11/2/024001.
- Nabuurs, G. J., et al. (2017) By 2050 the mitigation effects of EU forests could nearly double through climate smart forestry. Forests, 8, pp. 1–14.
- Walentowski, H., et al. (2017) Assessing future suitability of tree species under climate change by multiple methods: a case study in southern Germany. Annals of Forest Research, 60, pp. 101–126. doi.org/10.15287/afr.2016.789.
- Mäkelä, A., et al. (2000) Process-based models for forest ecosystem management: current state of the art and challenges for practical implementation. Tree Physiology, 20, pp. 289–298. doi.org/10.1093/treephys/20.5-6.289.
- Leech, S. M., et al., (2011) Assisted migration: adapting forest management to a changing climate. Journal of Ecosystems & Management, 12, pp. 18–34.
- Sass-Klaassen, U. G. W., et al. (2016) A tree-centered approach to assess impacts of extreme climatic events on forests. Frontiers in Plant Science, 7, p. 1069. doi.org/10.3389/fpls.2016.01069.
- Bowman, D. M. J. S., et al. (2013) Detecting trends in tree growth: not so simple. Trends in Plant Science, 18, pp. 11–17. doi.org/10.1016/j.tplants.2012.08.005.
- Hacket-Pain, A. J., et al. (2018) Climatically controlled reproduction drives interannual growth variability in a temperate tree species. Ecology Letters, 21, pp. 1833–1844. doi.org/10.1111/ele.13158.
- Dorji, Y., et al. (2019) Response of beech (Fagus sylvatica L.) trees to competition-new insights from using fractal analysis. Remote Sensing, 11, p. 2656. doi.org/10.3390/rs11222656.
- Petit-Cailleux, C., et al. (2019) Combining statistical and mechanistic models to unravel the drivers of mortality within a rear-edge beech population. bioRxiv. doi.org/10.1101/645747.
- Weigel, R., et al. (2019) Forest understory vegetation is more related to soil than to climate towards the cold distribution margin of European beech. Journal of Vegetation Science, 30, pp. 746–755. doi.org/10.1111/jvs.12759.
- Etzold, S., et al. (2020) Nitrogen deposition is the most important environmental driver of growth of pure, even-aged and managed European forests. Forest Ecology and Management, 458, p. 117762. doi.org/10.1016/j.foreco.2019.117762.
- Martínez-Sancho, E., et al. (2020) The GenTree dendroecological collection, tree-ring and wood density data from seven tree species across Europe. Scientific Data, 7, pp. 1–7. doi.org/10.1038/s41597-019-0340-y.
- Hartl-Meier, C., et al. (2014) Mountain forest growth response to climate change in the Northern Limestone Alps. Trees, 28, pp. 819–829. doi.org/10.1007/s00468-014-0994-1.
- Way, D. A. & Montgomery, R. A. (2015) Photoperiod constraints on tree phenology, performance and migration in a warming world. Plant Cell Environment, 38, pp. 1725–1736. doi.org/10.1111/pce.12431.
- Martínez del Castillo, E. et al. (2019) Spatial patterns of climate – growth relationships across species distribution as a forest management tool in Moncayo Natural Park (Spain). European Journal of Forest Research, 138, p. 299. doi.org/10.1007/s10342-019-01169-3.
- Hacket-Pain, A. J., et al. (2016). Consistent limitation of growth by high temperature and low precipitation from range core to southern edge of European beech indicates widespread vulnerability to changing climate. European Journal of Forest Research, 135, 897–909. doi.org/10.1007/s10342-016-0982-7
- van der Maaten, E. (2012) Climate sensitivity of radial growth in European beech (Fagus sylvatica L.) at different aspects in southwestern Germany. Trees, 26, pp. 777–788. doi.org/10.1007/s00468-011-0645-8.
- Decuyper, M. et al. (2020) Spatio-temporal assessment of beech growth in relation to climate extremes in Slovenia – an integrated approach using remote sensing and tree-ring data. Agricultural & Forest Meteorology, 287, p. 107925. doi.org/10.1016/j.agrformet.2020.107925.
- Kraus, C., (2016) Elevational response in leaf and xylem phenology reveals different prolongation of growing period of common beech and Norway spruce under warming conditions in the Bavarian Alps. European Journal of Forest Research, 135, pp. 1011–1023. doi.org/10.1007/s10342-016-0990-7.
- Martínez del Castillo, E. et al. (2016) Living on the edge: contrasted wood-formation dynamics in Fagus sylvatica and Pinus sylvestris under mediterranean conditions. Front. Plant Science, 7, p. 370. doi.org/10.3389/fpls.2016.00370.
- Čufar, K. et al. (2012) Temporal shifts in leaf phenology of beech (Fagus sylvatica) depend on elevation. Trees, 26, pp. 1091–1100. doi.org/10.1007/s00468-012-0686-7.
- Bontemps, J. D., et al. (2010) Dominant radial and height growth reveal comparable historical variations for common beech in north-eastern France. Forest Ecology and Management, 259, pp. 1455–1463. doi.org/10.1016/j.foreco.2010.01.019.
- Latte, N., et al. (2015) Increased tree-growth synchronization of beech (Fagus sylvatica L.) in response to climate change in northwestern Europe. Dendrochronologia, 33, pp. 69–77. doi.org/10.1016/j.dendro.2015.01.002.
- Zimmermann, J., et al. (2015) Climate warming-related growth decline affects Fagus sylvatica, but not other broad-leaved tree species in central european mixed forests. Ecosystems, 18, pp. 560–572. doi.org/10.1007/s10021-015-9849-x.
- Tegel, W., et al. (2014) A recent growth increase of European beech (Fagus sylvatica L.) at its Mediterranean distribution limit contradicts drought stress. European Journal of Forest Research, 133, pp. 61–71. doi.org/10.1007/s10342-013-0737-7.
- Hacket-Pain, A. J. & Friend, A. D. (2017) Increased growth and reduced summer drought limitation at the southern limit of Fagus sylvatica L., despite regionally warmer and drier conditions. Dendrochronologia, 44, pp. 22–30. doi.org/10.1016/j.dendro.2017.02.005.
- Dulamsuren, C., et al. (2017) European beech responds to climate change with growth decline at lower, and growth increase at higher elevations in the center of its distribution range (SW Germany). Trees, 31, pp. 673–686. doi.org/10.1007/s00468-016-1499-x.
- Spiecker, H., et al. (1996) Growth trends in European forests: studies from 12 countries. European Forest Institute Research Report.
- Cavin, L. & Jump, A. S. (2016) Highest drought sensitivity and lowest resistance to growth suppression are found in the range core of the tree Fagus sylvatica L. not the equatorial range edge. Global Change Biology, 23, pp. 1–18. doi.org/10.1111/gcb.13366.
- Mette, T. et al. (2013) Climatic turning point for beech and oak under climate change in Central Europe. Ecosphere, 4, pp. 1–19. doi.org/10.1890/ES13-00115.1.
- Michelot, A., et al. (2012) Comparing the intra-annual wood formation of three European species (Fagus sylvatica, Quercus petraea and Pinus sylvestris) as related to leaf phenology and non-structural carbohydrate dynamics. Tree Physiology, 32, pp. 1033–1045. doi.org/10.1093/treephys/tps052.
- Meier, I. C. & Leuschner, C. (2008) Belowground drought response of European beech: Fine root biomass and carbon partitioning in 14 mature stands across a precipitation gradient. Global Change Biology, 14, pp. 2081–2095. doi.org/10.1111/j.1365-2486.2008.01634.x.
- Leuschner, C. & Ellenberg, H. (2017) Ecology of Central European Forests. Vegetation Ecology of Central Europe. Vol. I: Springer.
- Allen, C. D., et al. (2015) On underestimation of global vulnerability to tree mortality and forest die-off from hotter drought in the Anthropocene. Ecosphere, 6, pp. 1–55. doi.org/10.1890/ES15-00203.1.
- Pechanec, V., et al. (2017) Ecological Informatics Modelling of the carbon sequestration and its prediction under climate change. doi.org/10.1016/j.ecoinf.2017.08.006.
- Speer, J H (2010) Fundamentals of Tree-Ring Research. University of Arizona Press.
- Biondi, F & Qeadan, F (2008) Removing the tree-ring width biological trend using expected basal area increment. in USDA Forest Service RMRS-P-55 124–131
- Karger, D. N. et al. (2017) Climatologies at high resolution for the earth’s land surface areas. Scientific Data, 4, pp. 1–20. doi.org/10.1038/sdata.2017.122.
- De Martonne, E (1926) Une nouvelle fonction climatologique: L’indice d’aridité. La Meteorology, 2, pp. 449–458.
- Martínez del Castillo, E., et al. (2019) errano-Notivoli, R. & de Luis, M. Modeling tree-growth: assessing climate suitability of temperate forests growing in Moncayo Natural Park (Spain). Forest Ecology and Management, 435, pp. 128–137. doi.org/10.1016/j.foreco.2018.12.051.
- Bolker, B. M. et al. (2009) Generalized linear mixed models: a practical guide for ecology and evolution. Trends in Ecology and Evolution, 24, pp. 127–135. doi.org/10.1016/j.tree.2008.10.008.
- Calcagno, V. & Mazancourt, C (2010) De. glmulti: an R package for easy automated model selection with (generalized) linear models. Journal of Statistical Software, 34(12), pp. 1–29. doi.org/10.18637/jss.v034.i12.
- Detry, M A & Ma Y (2016) Analyzing repeated measurements using mixed models. The Journal of the American Medical Association, 315, p. 407. doi.org/10.1001/jama.2015.19394.
- Harrison, X. A. et al. (2018) A brief introduction to mixed effects modelling and multi-model inference in ecology. PeerJ, pp. 1–32. doi.org/10.7717/peerj.4794.
- Johnson, J B & Omland, K S (2004) Model selection in ecology and evolution. Trends in Ecology & Evolution, 19, pp. 101–108. doi.org/10.1016/j.tree.2003.10.013.
- Caudullo, G., et al. (2017) Chorological maps for the main European woody species. Data in Brief, 12, pp. 662–666. https://doi.org/10.1016/j.dib.2017.05.007.
- Meinshausen, M. et al. (2020) The shared socio-economic pathway (SSP) greenhouse gas concentrations and their extensions to 2500. Geoscientific Model Development, 13, pp. 3571–3605. https://doi.org/10.5194/gmd-13-3571-2020.
- Karger, D N & Zimmermann, N E (2018) CHELSAcruts - High Resolution Temperature And Precipitation Timeseries For The 20th Century And Beyond. https://doi.org/10.16904/envidat.159
- Norinder, U., et al. (2016) Conformal prediction to define applicability domain - a case study on predicting ER and AR binding. SAR and QSAR in Environmental Research, 27, pp. 303–316. doi.org/10.1080/1062936X.2016.1172665.
- Metzger, M. J., et al. (2005) A climatic stratification of the environment of Europe. Global Ecology and Biogeography, 14, pp. 549–563. doi.org/10.1111/j.1466-822X.2005.00190.x