Due to its deep modification of biotic and abiotic elements compared to natural habitats, the urban environment, which is extending every year with human population expansion, causes problems for bird survival.
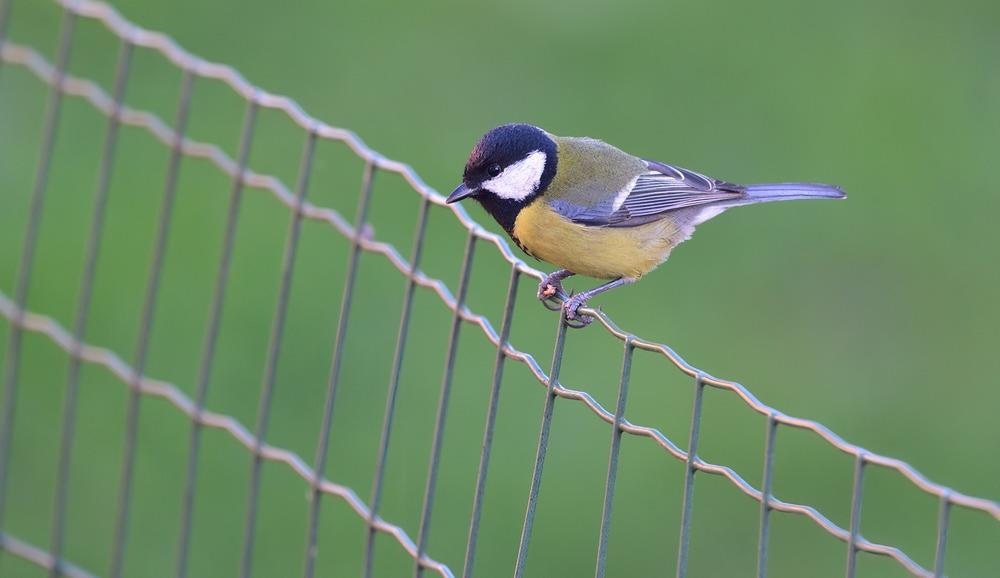
Image Credit: Davide Bonora/Shutterstock.com
Urbanization causes habitat fragmentation and a major reduction in vegetative cover, as well as increased lighting duration and sound levels, a rise in local temperature, and chronic exposure to a variety of hazardous chemical substances.
In metropolitan individuals, physiological and genetic changes are also documented, and various studies have indicated environmental pressures on individual health and survival.
The first goal of recent research published in Birds was to see if the urban environment has a filtering effect on the quality of life of the birds living there.
The study’s second goal was to see if there were any reproductive consequences in terms of physical condition and telomere length by collecting longitudinal data from birds captured in the winter and recaptured throughout the breeding season. Researchers expected a considerable decrease in the physical condition and telomere length in the urban location than in the forest site due to higher reproductive limitations in the city.
Methodology
This research, which took place between February and June 2019, is part of long-term research on Great Tits populations living in artificial nest boxes along an urbanization gradient in Strasbourg, France.
The Great Tit is a popular passerine that breeds well in artificial nest boxes, making reproduction and adult captures easier to track. Furthermore, this species is an urban adaptor, able to survive in both human and natural environments.
Between April and June, Great Tits breed and lay an average of 7.5 eggs in the city and 9 eggs in the forest, which are incubated for about 13 days. A forest population residing in La Wantzenau forest within 12-20 km of Strasbourg was tracked, as was an urban population living in various urban parks and tree-lined walkways in the central region of Strasbourg.
Individuals from both sites were captured twice: first in the winter after the birds had dispersed but before breeding and again during the breeding season.
Results and Discussion
Regardless of the season or location, the age of collected birds differed significantly by sex. Females were taken at a younger age than males. None of the evaluated variables were significant when it came to body conditions (Table 1).
Table 1. Result of statistical analyses of morphological and genomic parameters between urban and forest birds and between seasons (winter and reproduction). Source: Saulnier, et al., 2022
|
Age (n = 161) |
Body Size Index (n = 161) |
Body Condition (n = 161) |
RTL (n = 151) |
Variable |
Chi² |
df |
p |
F |
df |
p |
F |
df |
p |
F |
df |
p |
Site |
0.156 |
1, 154 |
0.693 |
45.66 |
1, 153 |
<0.001 |
0.6002 |
1, 149 |
0.440 |
0.548 |
1, 142 |
0.461 |
Sex |
61.35 |
1, 154 |
<0.001 |
39.97 |
1, 153 |
<0.001 |
1.6297 |
1, 149 |
0.203 |
0.395 |
1, 142 |
0.531 |
Season |
0.400 |
1, 154 |
0.527 |
4.46 |
1, 153 |
0.042 |
3.9457 |
1, 149 |
0.051 |
0.623 |
1, 142 |
0.432 |
Age |
|
|
|
5.82 |
1, 153 |
0.017 |
1.1800 |
1, 149 |
0.280 |
0.812 |
1, 142 |
0.370 |
Body condition |
|
|
|
|
|
|
|
|
|
0.577 |
1, 142 |
0.450 |
Site × Age |
|
|
|
|
|
|
|
|
|
|
|
|
Site × Season |
|
|
|
4.18 |
1, 153 |
0.048 |
|
|
|
4.525 |
1, 142 |
0.037 |
Site × Sex |
|
|
|
|
|
|
|
|
|
|
|
|
A substantial connection between the season and the site was discovered for the body size index (Table 1, Figure 1).
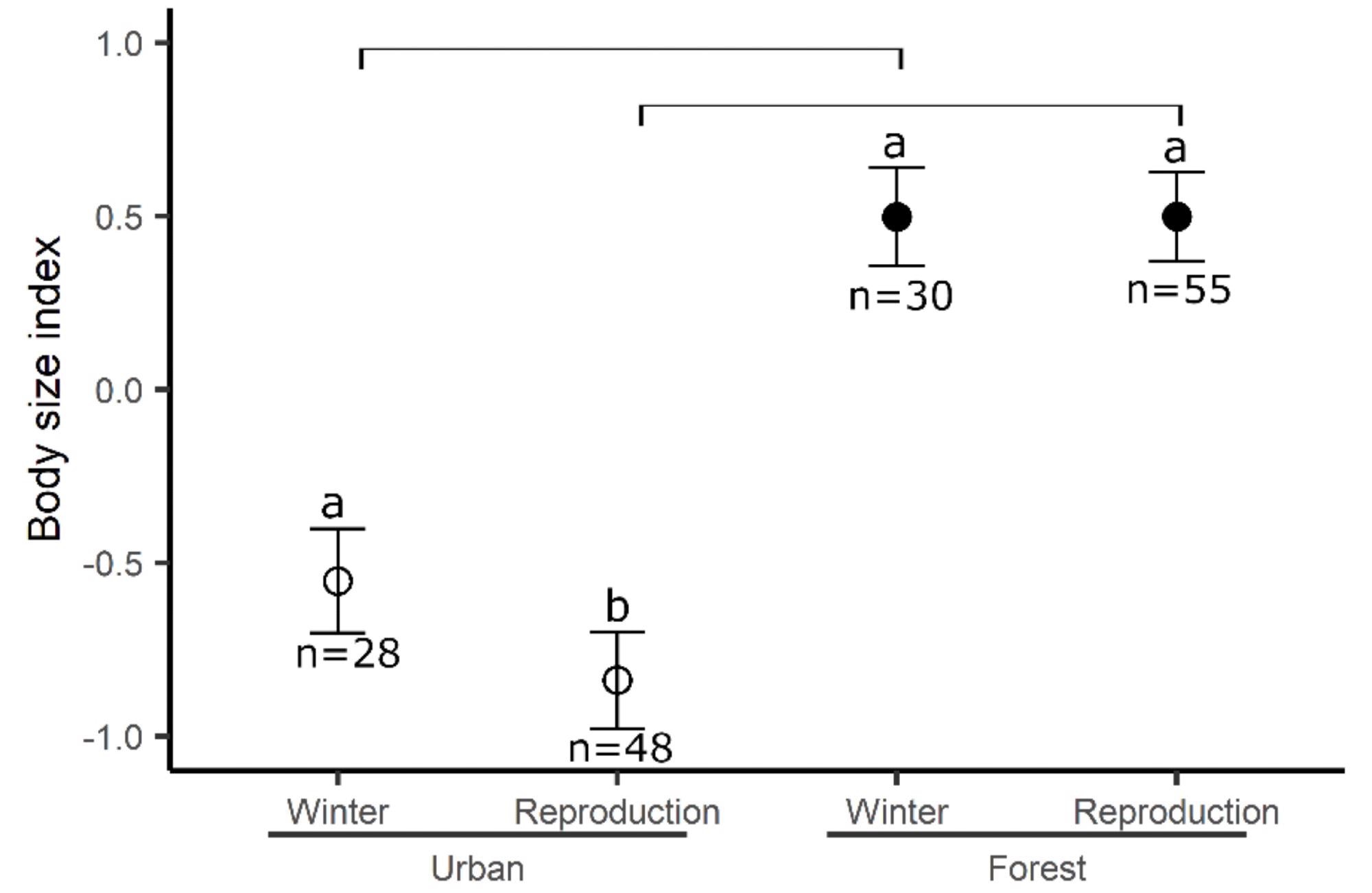
Figure 1. The body size of birds between sites across seasons. Means and standard errors are represented. Different letters represent significant differences between seasons for the same site and bars represent the difference between sites for the same season. Image Credit: Source: Saulnier, et al., 2022
For RTL, the interplay between the season and the location was equally important (Figure 2). Winter telomeres in urban birds were longer than telomeres in urban birds taken during reproduction and winter telomeres in forest birds.
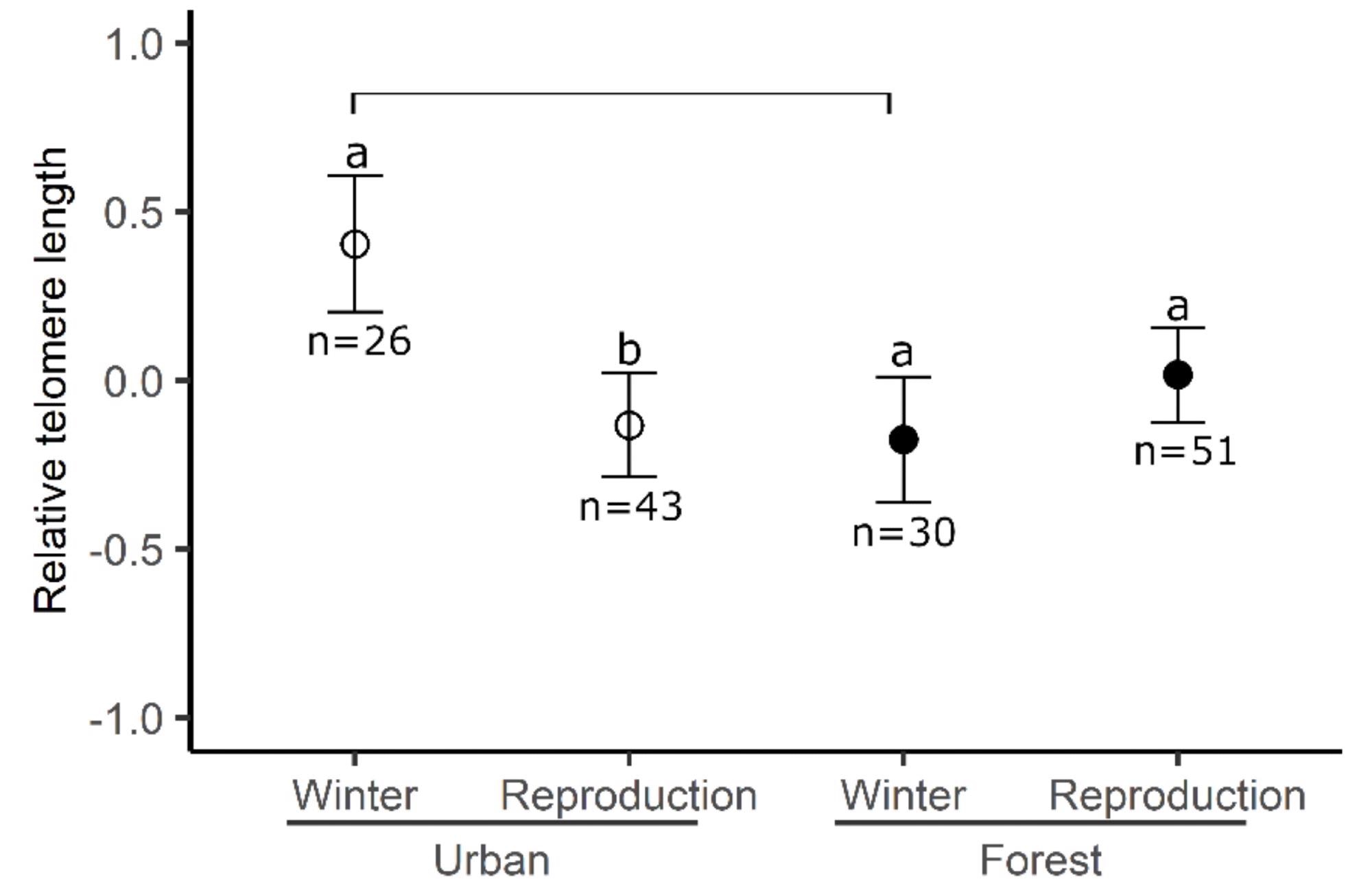
Figure 2. The relative telomere length of birds between sites across seasons. Means and standard errors are represented. Different letters represent significant differences between seasons for the same site and bars represent the difference between sites for the same season. Image Credit: Source: Saulnier, et al., 2022
Finally, researchers looked at differences in the physical condition and RTL of birds caught over both seasons. Between winter and reproduction, none of the variables studied substantially explained changes in body condition or RTL (Table 2).
Table 2. Result of statistical analyses of morphological and genomic parameters changes between seasons for re-caught birds. Source: Saulnier, et al., 2022
|
Body Condition (n = 27) |
RTL (n = 23) |
Variable |
F |
df |
p |
F |
df |
p |
Site |
0.048 |
1, 21 |
0.829 |
0.161 |
1, 16 |
0.694 |
Sex |
0.523 |
1, 21 |
0.478 |
1.105 |
1, 16 |
0.310 |
Age |
2.287 |
1, 21 |
0.146 |
0.069 |
1, 16 |
0.796 |
Fledging number |
0.850 |
1, 21 |
0.367 |
0.689 |
1, 16 |
0.419 |
Hatching date |
0.111 |
1, 21 |
0.742 |
2.778 |
1, 16 |
0.115 |
RTL Winter |
|
|
|
0.527 |
1, 16 |
0.479 |
There were no variations in body condition between sites or seasons. Due to the high energetic demands associated with reproduction, bodily condition before breeding is linked to breeding success.
Nonetheless, investigations on urbanization in different bird species, particularly in adult birds, have not consistently found a difference in bodily condition. Anthropogenic food, which is more predictable and abundant, compensates for the loss of prey in cities.
Human-provided diets, on the other hand, are frequently of lower quality than natural foods, often providing insufficient protein, and can result in physiological deficits.
The findings also show a favorable link between body size and avian age, regardless of size or season. This connection could be the consequence of feather quality and the molt stage of the birds, as the body size index is partly linked with wing size.
In a study of common blackbirds, a relationship between wing lengths and age was discovered: younger blackbirds tended to have shorter wings than older birds.
Feather grade and length tend to rise between the post-fledging partial molt and the post-breeding adult molts for a variety of passerine species, particularly Great Tits. However, there was no change in age distribution between sites or seasons, implying that smaller breeders exist regardless of the age of the birds.
To fully comprehend the links between telomere length, reproductive costs, and weather in the city, it would be fascinating to conduct this experiment over several years with varying weather conditions.
Bolder birds had lower maximum corticosterone concentrations, stronger antioxidant capacity, and lower concentrations of reactive oxygen metabolites, resulting in decreased oxidative stress, according to certain research. The fact that urban birds have longer telomeres in the winter could be related to the selection of birds with a stronger ability for self-maintenance or a lower metabolic cost, which affects telomeres.
Similarly, trap feeders were placed near artificial food sources, which are an important element of urban birds’ winter diet. It is possible that larger individuals, who have higher energy needs, prefer these areas more than smaller individuals, increasing their chances of being caught.
Conclusion
Finally, the findings show morphological and genetic differences between winter individuals and breeders, but the topic of the city’s faltering role remains unanswered. If researchers consider that a smaller size offers an advantage in an urban setting and that smaller persons are of higher quality, researchers can conclude that the urban environment does indeed filter individual quality.
The results for telomere length throughout the reproduction period, on the other hand, do not support the filter hypothesis. These findings point to a greater cost of reproduction in the metropolis that is influenced by weather conditions.
Long-term research is needed to better comprehend the selection mechanisms that occur in cities, as well as the dynamics connected with inter-annual weather fluctuations.
Journal Reference:
Saulnier, A., Bleu, J., Lemonnier, G., Uhlrich, P., Zahn, S., Massemin, S. (2022) Does the Urban Environment Act as a Filter on the Individual Quality of Birds? Birds, 3(1), pp. 84-98. Available Online: https://www.mdpi.com/2673-6004/3/1/7/htm.
References and Further Reading
- Stearns, S C (1992) The Evolution of Life Histories; Oxford University Press: Oxford, UK.
- Lailvaux, S P & Husak, J F (2014) The life history of whole-organism performance. The Quarterly Review of Biology, 89, pp. 285–318. doi.org/10.1086/678567.
- McNamara, J M & Houston, A I (1996) State-dependent life histories. Nature, 380, pp. 215–221. doi.org/10.1038/380215a0.
- Sudyka, J (2019) Does reproduction shorten telomeres? Towards integrating individual quality with life-history strategies in telomere biology. BioEssays, 41, p. 1900095. doi.org/10.1002/bies.201900095.
- Wilson, A J & Nussey, D H (2010) What is individual quality? An evolutionary perspective. Trends in Ecology Evolution, 25, pp. 207–214. doi.org/10.1016/j.tree.2009.10.002.
- Blums, P., et al. (2005) Individual quality, survival variation and patterns of phenotypic selection on body condition and timing of nesting in birds. Oecologia, 143, pp. 365–376. doi.org/10.1007/s00442-004-1794-x.
- Vedder, O & Bouwhuis, S (2018) Heterogeneity in individual quality in birds: Overall patterns and insights from a study on common terns. Oikos, 127, pp. 719–727. doi.org/10.1111/oik.04273.
- Peralta-Sánchez, J. M., et al. (2020) Females are more determinant than males in reproductive performance in the house sparrow Passer domesticus. Journal of Avian Biology, 51, e02240.
- Dukas, R (2019) Animal expertise: Mechanisms, ecology and evolution. Animal Behaviour, 147, pp. 199–210. doi.org/10.1016/j.anbehav.2018.05.010.
- Forslund, P & Pärt, T (1995) Age and reproduction in birds—Hypotheses and tests. Trends in Ecology Evolution, 10, pp. 374–378. doi.org/10.1016/S0169-5347(00)89141-7.
- Pitera, A. M., et al. (2021) Reproduction is affected by individual breeding experience but not pair longevity in a socially monogamous bird. Behavioral Ecology and Sociobiology, 75, pp. 1–11. doi.org/10.1007/s00265-021-03042-z.
- Espie, R. H. M., et al. (2000) Age-dependent breeding performance in Merlins (Falco columbarius). Ecology, 81, pp. 3404–3415. doi.org/10.2307/177503.
- Hamman, J & Cooke, F (2008) Age effects on clutch size and laying dates of individual female Lesser Snow Geese Anser caerulescens. Ibis, 129, pp. 527–532. doi.org/10.1111/j.1474-919X.1987.tb08240.x.
- Wiktander, U., et al. (2001) Age and Reproduction in Lesser Spotted Woodpeckers (Dendrocopos minor). The Auk, 118, pp. 624–635. doi.org/10.1093/auk/118.3.624.
- Hatch, M I & Westneat, D F (2007) Age-related patterns of reproductive success in house sparrows Passer domesticus. Journal of Avian Biology, 38, pp. 603–611. doi.org/10.1111/j.0908-8857.2007.04044.x.
- Riechert, J., et al. (2012) Why do experienced birds reproduce better? Possible endocrine mechanisms in a long-lived seabird, the common tern. General and Comparative Endocrinology, 178, pp. 391–399. doi.org/10.1016/j.ygcen.2012.06.022.
- Isaksson, C & Andersson, S (2007) Carotenoid diet and nestling provisioning in urban and rural Great Tits Parus major. Journal of Avian Biology, 38, pp. 564–572. doi.org/10.1111/j.2007.0908-8857.04030.x.
- Biard, C., et al. (2017) Growing in cities: An urban penalty for wild birds? A study of phenotypic differences between urban and rural Great Tit chicks (Parus major). Frontiers in Ecology and Evolution, 5, pp. 1–14. https://doi.org/10.3389/fevo.2017.00079.
- Vaugoyeau, M., et al. (2016) Interspecific variation in the relationship between clutch size, laying date and intensity of urbanization in four species of hole-nesting birds. Ecology and Evolution, 6, pp. 5907–5920. doi.org/10.1002/ece3.2335.
- Gil, D & Brumm, H (2013) Avian Urban Ecology; Oxford University Press: Oxford, UK, ISBN 9780199661572.
- Jones, E L & Leather, S R (2012) Invertebrates in urban areas: A review. European Journal of Entomology, 109, pp. 463–478. doi.org/10.14411/eje.2012.060.
- Fenoglio, M. S., et al. (2020) Negative effects of urbanization on terrestrial arthropod communities: A meta-analysis. Global Ecology and Biogeography, 29, pp. 1412–1429. doi.org/10.1111/geb.13107.
- MacLeod, R., et al. (2006) Mass-dependent predation risk as a mechanism for house sparrow declines? Biology Letters, 2, pp. 43–46. doi.org/10.1098/rsbl.2005.0421.
- Marzluff, J M (2001) Worldwide urbanization and its effects on birds. In Avian Ecology and Conservation in an Urbanizing World; Springer: Boston, MA, USA, pp. 19–47. doi.org/10.1007/978-1-4615-1531-9_2.
- Sorace, A & Gustin, M (2009) Distribution of generalist and specialist predators along urban gradients. Landscape and Urban Planning, 90, pp. 111–118. doi.org/10.1016/j.landurbplan.2008.10.019.
- Rodewald, A D & Kearns, L J (2011) Shifts in dominant nest predators along a rural-to-urban landscape gradient. The Condor, 113, pp. 899–906. doi.org/10.1525/cond.2011.100132.
- Van Heezik, Y., et al. (2010) Do domestic cats impose an unsustainable harvest on urban bird populations? Biological Conservation, 143, pp. 121–130. doi.org/10.1016/j.biocon.2009.09.013.
- Chamberlain, D. E., et al. (2009) Avian productivity in urban landscapes: A review and meta-analysis. Ibis, 151, pp. 1–18. doi.org/10.1111/j.1474-919X.2008.00899.x.
- Sepp, T., et al. (2018) A review of urban impacts on avian life-history evolution: Does city living lead to slower pace of life? Global Change Biology, 24, pp. 1452–1469. doi.org/10.1111/gcb.13969.
- Wawrzyniak, J., et al. (2020) Differences in the breeding performance of Great Tits Parus major between a forest and an urban area: A long term study on first clutches. The European Zoological Journal, 87, pp. 294–309. doi.org/10.1080/24750263.2020.1766125.
- Halfwerk, W., et al. (2011) Negative impact of traffic noise on avian reproductive success. Journal Applied Ecology, 48, pp. 210–219. doi.org/10.1111/j.1365-2664.2010.01914.x.
- Bailly, J., et al. (2016) From eggs to fledging: Negative impact of urban habitat on reproduction in two tit species. Journal of Ornithology, 157, pp. 377–392. doi.org/10.1007/s10336-015-1293-3.
- Peach, W. J., et al. (2015) Invertebrate prey availability limits reproductive success but not breeding population size in suburban House Sparrows Passer domesticus. Ibis, 157, pp. 601–613. doi.org/10.1111/ibi.12264.
- Bailly, J., et al. (2017) Multi-element analysis of blood samples in a passerine species: Excesses and deficiencies of trace elements in an urbanization study. Frontiers in Ecology and Evolution, 5, p. 6. doi.org/10.3389/fevo.2017.00006.
- Isaksson, C., et al. (2008) Egg yolk carotenoids in relation to habitat and reproductive investment in the Great Tit Parus major. Physiological and Biochemical Zoology, 81, pp. 112–118. doi.org/10.1086/522650.
- Jarrett, C., et al. (2020) Bitter fruits of hard labour: Diet metabarcoding and telemetry reveal that urban songbirds travel further for lower-quality food. Oecologia, 193, pp. 377–388. doi.org/10.1007/s00442-020-04678-w.
- Hinsley, S. A., et al. (2008) Effects of structural and functional habitat gaps on breeding woodland birds: Working harder for less. Landscape Ecology, 23, pp. 615–626. doi.org/10.1007/s10980-008-9225-8.
- Estes, W A & Mannan, R W (2003) Feeding behavior of cooper’s hawks at urban and rural nests in southeastern arizona. The Condor, 105, pp. 107–116. doi.org/10.1093/condor/105.1.107.
- Blair, R B (1996) Land use and avian species diversity along an urban gradient. Ecological Applications, 6, pp. 506–519. doi.org/10.2307/2269387.
- Clergeau, P., et al. (2006) Avifauna homogenisation by urbanisation: Analysis at different European latitudes. Biological Conservation, 127, pp. 336–344. doi.org/10.1016/j.biocon.2005.06.035.
- McKinney, M L (2006) Urbanization as a major cause of biotic homogenization. Biological Conservation, 127, pp. 247–260. doi.org/10.1016/j.biocon.2005.09.005.
- Croci, S., et al. (2008) Does urbanization filter birds on the basis of their biological traits? The Condor, 110, pp. 223–240. doi.org/10.1525/cond.2008.8409.
- Kurucz, K., et al. (2021) Urbanization shapes bird communities and nest survival, but not their food quantity. Global Ecology and Conservation, 26, p. e01475. doi.org/10.1016/j.gecco.2021.e01475.
- Liker, A., et al. (2008) Lean birds in the city: Body size and condition of house sparrows along the urbanization gradient. Journal of Animal Ecology, 77, pp. 789–795. doi.org/10.1111/j.1365-2656.2008.01402.x.
- Meillère, A., et al. (2015) Influence of urbanization on body size, condition, and physiology in an urban exploiter: A multi-component approach. PLoS ONE, 10, pp. 1–19. doi.org/10.1371/journal.pone.0135685.
- Evans, K. L., et al. (2009) The effect of urbanisation on avian morphology and latitudinal gradients in body size. Oikos, 118, pp. 251–259. doi.org/10.1111/j.1600-0706.2008.17092.x.
- Shochat, E (2004) Credit or debit? Resource input changes population dynamics of city-slicker birds. Oikos, 106, pp. 622–626. doi.org/10.1111/j.0030-1299.2004.13159.x.
- Møller, A P (2009) Successful city dwellers: A comparative study of the ecological characteristics of urban birds in the Western Palearctic. Oecologia, 159, pp. 849–858. doi.org/10.1007/s00442-008-1259-8.
- Caizergues, A. E., et al. (2021) An avian urban morphotype: How the city environment shapes Great Tit morphology at different life stages. Urban Ecosystem, 24, pp. 929–941. doi.org/10.1007/s11252-020-01077-0.
- Meillère, A., et al. (2017) Growing in a city: Consequences on body size and plumage quality in an urban dweller, the house sparrow (Passer domesticus). Landscape and Urban Planning, 160, pp. 127–138. doi.org/10.1016/j.landurbplan.2016.12.014.
- Milenkaya, O., et al. (2015) Body condition indices predict reproductive success but not survival in a sedentary, tropical bird. PLoS ONE, 10, p. e0136582. doi.org/10.1371/journal.pone.0136582.
- Lamb, J. S., et al. (2016) Physical condition and stress levels during early development reflect feeding rates and predict pre-And post-fledging survival in a nearshore seabird. Conservation Physiology, 4, p. cow060. doi.org/10.1093/conphys/cow060.
- Hõrak, P., et al. (1999) Immune function and survival of Great Tit nestlings in relation to growth conditions. Oecologia, 121, pp. 316–322. doi.org/10.1007/s004420050934.
- Blackburn, E H (1991) Structure and function of telomeres. Nature, 350, pp. 569–573. doi.org/10.1038/350569a0.
- Blackburn, E H (2005) Telomeres and telomerase: Their mechanisms of action and the effects of altering their functions. FEBS Letters, 579, pp. 859–862. doi.org/10.1016/j.febslet.2004.11.036.
- Tricola, G. M., et al. (2018) The rate of telomere loss is related to maximum lifespan in birds. Philosophical Transactions of the Royal Society B: Biological Sciences, 373, p. 20160445. doi.org/10.1098/rstb.2016.0445.
- Haussmann, M F & Mauck, R A (2008) Telomeres and longevity: Testing an evolutionary hypothesis. Molecular Biology and Evolution, 25, PP. 220–228. doi.org/10.1093/molbev/msm244.
- Monaghan, P., et al. (2009) Oxidative stress as a mediator of life history trade-offs: Mechanisms, measurements and interpretation. Ecology Letters, 12, pp. 75–92. doi.org/10.1111/j.1461-0248.2008.01258.x.
- Stauffer, J., et al. (2017) Telomere damage and redox status alterations in free-living passerines exposed to metals. Science of The Total Environment, 575, pp. 841–848. doi.org/10.1016/j.scitotenv.2016.09.131.
- Blévin, P., et al. (2017) Perfluorinated substances and telomeres in an Arctic seabird: Cross-sectional and longitudinal approaches. Environmental Pollution, 230, pp. 360–367. doi.org/10.1016/j.envpol.2017.06.060.
- Meillère, A., et al. (2015) Traffic noise exposure affects telomere length in nestling house sparrows. Biology Letters, 11, p. 20150559. doi.org/10.1098/rsbl.2015.0559.
- Grunst, A. S., et al. (2020) Proximity to roads, but not exposure to metal pollution, is associated with accelerated developmental telomere shortening in nestling Great Tits. Environmental Pollution, 256, p. 113373. doi.org/10.1016/j.envpol.2019.113373.
- Young, R. C., et al. (2017) Effects of developmental conditions on growth, stress and telomeres in black-legged kittiwake chicks. Molecular Ecology, 26, pp. 3572–3584. doi.org/10.1111/mec.14121.
- Boonekamp, J. J., et al. (2017) Does oxidative stress shorten telomeres? Biology Letters, 13, p. 20170164. doi.org/10.1098/rsbl.2017.0164.
- Chatelain, M., et al. (2020) The association between stressors and telomeres in non-human vertebrates: A meta-analysis. Ecology Letters, 23, pp. 381–398. doi.org/10.1111/ele.13426.
- Epel, E. S., et al. (2004) Accelerated telomere shortening in response to life stress. Proceedings of the National Academy of Sciences of the United States of America, USA, 101, pp. 17312–17315. doi.org/10.1073/pnas.0407162101.
- Kim, S Y & Velando, A (2015) Antioxidants safeguard telomeres in bold chicks. Biology Letters, 11, p. 20150211. doi.org/10.1098/rsbl.2015.0211.
- Haussmann, M. F., et al. (2005) Longer telomeres associated with higher survival in birds. Biology Letters, 1, pp. 212–214. doi.org/10.1098/rsbl.2005.0301.
- Ibáñez-Álamo, J. D., et al. (2018) Urban blackbirds have shorter telomeres. Biology Letters, 14, p. 20180083. doi.org/10.1098/rsbl.2018.0083.
- Salmón, P., et al. (2017) Selective disappearance of Great Tits with short telomeres in urban areas. Proceedings of the Royal Society B: Biological Sciences, 284, p. 20171349. doi.org/10.1098/rspb.2017.1349.
- Criscuolo, F., et al. (2009) Real-time quantitative PCR assay for measurement of avian telomeres. Journal of Avian Biology, 40, 342–347. doi.org/10.1111/j.1600-048X.2008.04623.x.
- R Development Core Team (2021) R: A Language and Environment for Statistical Computing; R Foundation for Statistical Computing, Ed.; R Development Core Team: Vienna, Austria, ISBN 3-900051-07-0.
- Verhulst, S (2020) Improving comparability between qPCR-based telomere studies. Molecular Ecology Resources, 20, pp. 11–13. doi.org/10.1111/1755-0998.13114.
- Labocha, M K & Hayes, J P (2012) Morphometric indices of body condition in birds: A review. Journal of Ornithology, 153, pp. 1–22. doi.org/10.1007/s10336-011-0706-1.
- Hayes, J P & Shonkwiler, J S (2001) Morphological indicators of body condition: Useful or wishful thinking? Body Composition Analysis of Animals, 10, pp. 8–38. doi.org/10.1017/CBO9780511551741.003.
- Bates, D., et al. (2015) Fitting linear mixed-effects models using lme4. Journal of Statistical Software, 67, pp. 1–48. doi.org/10.18637/jss.v067.i01.
- Chastel, O., et al. (1995) Influence of body condition on reproductive decision and reproductive success in the Blue Petrel. The Auk, 112, pp. 964–972. doi.org/10.2307/4089027.
- Isaksson, C., et al. (2017) Species-dependent effects of the urban environment on fatty acid composition and oxidative stress in birds. Frontiers in Ecology and Evolution, 5, pp. 1–13. doi.org/10.3389/fevo.2017.00044.
- Corsini, M., et al. (2020) Growing in the city: Urban evolutionary ecology of avian growth rates. Evolutionary Applications, 14, pp. 69–84. doi.org/10.1111/eva.13081.
- Caizergues, A. E., et al. (2018) Urban versus forest ecotypes are not explained by divergent reproductive selection. Proceedings B is the Royal Society's, 285, p. 20180261. doi.org/10.1098/rspb.2018.0261.
- McDonnell, M J & Hahs, A K (2015) Adaptation and Adaptedness of Organisms to Urban Environments. Annual Review of Ecology, Evolution, and Systematics, 46, pp. 261–280. doi.org/10.1146/annurev-ecolsys-112414-054258.
- Hedenstrom, A & Møller, A P (1992) Morphological adaptations to song flight in passerine birds: A comparative study. Proceedings of the Royal Society of London. Series B, Biological Sciences, 247, pp. 183–187. doi.org/10.1098/rspb.1992.0026.
- Møller, A P (2008) Flight distance of urban birds, predation, and selection for urban life. Behavioral Ecology and Sociobiology, 63, pp. 63–75. doi.org/10.1007/s00265-008-0636-y.
- Gosler, A. G., et al. (1995) Predation risk and the cost of being fat. Nature, 377, pp. 621–623. doi.org/10.1038/377621a0.
- Kiat, Y & Sapir, N (2018) Life-history trade-offs result in evolutionary optimization of feather quality. Biological Journal of the Linnean Society, 125, pp. 613–624. doi.org/10.1093/biolinnean/bly135.
- Pap, P. L., et al. (2007) Increase of feather quality during moult: A possible implication of feather deformities in the evolution of partial moult in the Great Tit Parus major. Journal of Avian Biology, 38, pp. 471–478. doi.org/10.1111/j.0908-8857.2007.03958.x.
- Giraudeau, M., et al. (2019) Do telomeres influence pace-of-life-strategies in response to environmental conditions over a lifetime and between generations? BioEssays, 41, p. 1800162. doi.org/10.1002/bies.201800162.
- Heidinger, B. J., et al. (2012) Telomere length in early life predicts lifespan. Proceedings of the National Academy of Sciences of the United States of America. USA, 109, pp. 1743–1748. doi.org/10.1073/pnas.1113306109.
- Bauch, C., et al. (2013) Telomere length reflects phenotypic quality and costs of reproduction in a long-lived seabird. Proceedings of the Royal Society of London. Series B, Biological Sciences, 280, p. 20122540. doi.org/10.1098/rspb.2012.2540.
- Angelier, F., et al. (2019) Is telomere length a molecular marker of individual quality? Insights from a long-lived bird. Functional Ecology, 33, pp. 1076–1087. doi.org/10.1111/1365-2435.13307.
- Krijgsveld, K. L., et al. (2003) Foraging behavior and physiological changes in precocial quail chicks in response to low temperatures. Physiology & Behavior, 79, pp. 311–319. doi.org/10.1016/S0031-9384(03)00117-3.
- Avery, M I & Krebs, J R (1984) Temperature and foraging success of Great Tits Parus major hunting for spiders. Ibis, 126, pp. 33–38. doi.org/10.1111/j.1474-919X.1984.tb03661.x.
- Atwell, J. W., et al. (2012) Boldness behavior and stress physiology in a novel urban environment suggest rapid correlated evolutionary adaptation. Behavioral Ecology, 23, pp. 960–969. doi.org/10.1093/beheco/ars059.
- Herborn, K. A., et al. (2011) Oxidative profile varies with personality in European greenfinches. The Journal of Experimental Biology, 214, pp. 1732–1739. doi.org/10.1242/jeb.051383.