Decomposition and humification are two continuous mechanisms that decide the fate of plant litter, but they are currently largely uncoupled in research. Litter decomposition has now become ever more well understood in recent years, but the humification process is still poorly understood. This article looks at current research published in Forests.
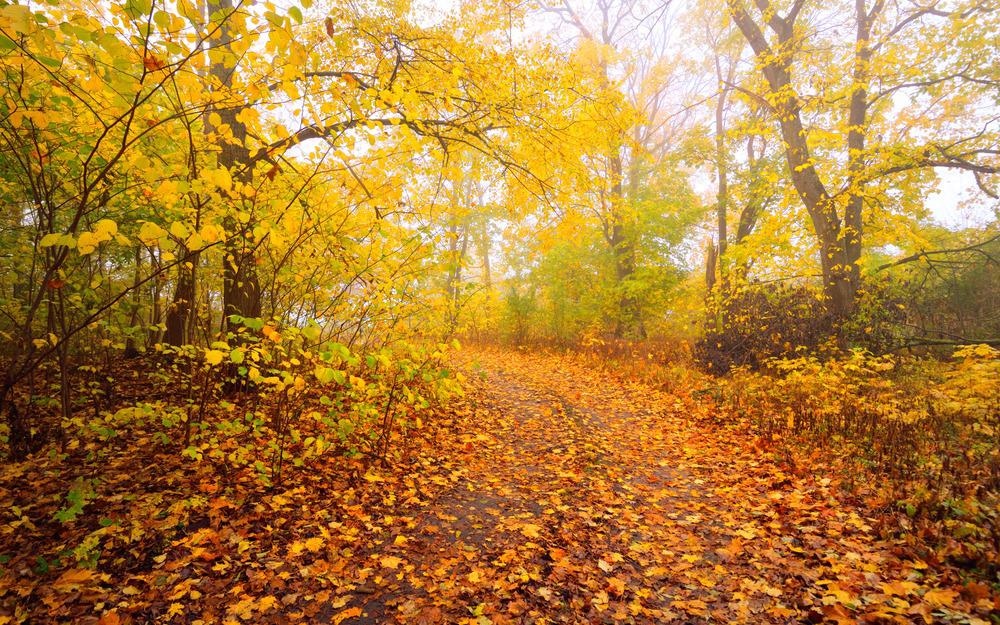
Image Credit: Alex Stemmers/Shutterstock.com
According to one theory, litter decomposes to a “limit value,” and recalcitrant residues that cannot decompose are polymerized into humus. As a result, humus is thought to collect at the end of the litter decomposition process. Current evidence, however, contradicts this long-held belief, demonstrating that significant litter carbon is deposited in mineral soil all through early litter decay.
The International Humic Substances Society’s alkali extraction method’s humic acid (HA) and fulvic acid (FA) fractions still provide historical comparative data and accurately depict natural organic matter all over multiple environments, source materials, and research objectives.
Although these isotope-labeling experiments have greatly improved the understanding of carbon flux from plant litter to soils, the processes by which humus gathers in the decomposing litter substrate remain unclear.
In the Northern Hemisphere, snow covers half of the land area and functions as a thermal insulator. Seasonal snow cover, on the other hand, has lowered by 7% and is expected to decrease by 25% by the end of the century. It has been demonstrated that a decrease in snow cover slows the carbon flux from terrestrial plants to the soil via litter decomposition.
As the preliminary findings show, lowered snow cover may have a significant impact on litter humification, which sequesters carbon in the litter that is decomposing, rather than litter decomposition, which enables the litter to decompose in cold forest ecosystems.
The current research answers two questions:
- Is humus created at the end of the litter decomposition process?
- Will the carbon sequestration process in high-altitude ecosystems be affected by lowered snow cover?
The overall goal was to learn more about how plant litter decomposes and becomes humified after leaf fall.
In 2012, an in situ litterbag experiment was carried out in an alpine forest on the eastern Tibetan Plateau to investigate the chemical changes, humus accumulation, elemental dynamics, and microbial community during the decomposition and humification of one twig litter and six foliar litters, which were used to reduce litter species and types uncertainty.
Because winter litter decomposition is a major ecological process in this alpine forest, samplings were planned at the end of snow formation, coverage, and melt stages in winter, as well as at the end of the growing season (Table 1), with an importance placed on wintertime to investigate the temporal effects of reduced snow cover.
Table 1. Sampling compared with the timings and lengths of winters during the four years of decomposition. Hourly temperature with a 50% probability (continual 12 hours per day) of freezing (below 0 °C) is defined as the beginning and end of winter in this study (modified from Ladwig et al., 2016). This definition is not conservative because solar radiation is very strong in this alpine forest and increases in temperature were recorded using a data logger. Sampling dates were scheduled approximately in late April and October within one-week intervals referring to the timing of the 2012/2013 winter. Source: Wang et al., 2022
Winter |
Timing of Winter |
Length of Winter (Days) |
Sampling Date |
Beginning |
End |
End of Growing Season |
End of Snowmelt |
2012/2013 winter |
30 October 2012 |
24 April 2013 |
176 |
Experiment began |
24 April 2013 |
2013/2014 winter |
31 October 2013 |
30 April 2014 |
181 |
30 October 2013 |
24 April 2014 |
2014/2015 winter |
29 October 2014 |
26 April 2015 |
179 |
29 October 2014 |
23 April 2015 |
2015/2016 winter |
30 October 2015 |
26 April 2016 |
179 |
2 November 2015 |
24 April 2016 |
The current article presents data on humus content (alkali-extractable substances such as fulvic acid and humic acid) in three tree and two shrub foliar litters with varying initial qualities (Table 2) over four years (2012–2016) and its response to snow cover reduction. The findings point to a potential long-term consequence of SOM formation with lowered winter snow cover in a changing climate.
Table 2. Initial chemical compounds in the five foliar litters used in the long-term decomposition experiment. Data from Ni et al., 2015;2016. Values are means and standard deviations in parentheses (n = 3). Different superscript letters in the same column represent significant (p < 0.05) differences between litter species. C: carbon, N: nitrogen, P: phosphorus, WSS: water-soluble substances, OSS: organic-soluble substances, ASS: acid-soluble substances, AUR: acid-unhydrolyzable residues. Source: Wang et al., 2022
Litter |
C (%) |
N (%) |
P (%) |
WSS (%) |
OSS (%) |
ASS (%) |
AUR (%) |
C/N Ratio |
AUR/N Ratio |
Cypress |
51.64 (1.77) a |
0.88 (0.010) c |
0.12 (0.006) ab |
35.74 (0.69) c |
33.16 (3.43) a |
32.43 (1.29) a |
20.60 (3.41) b |
58.86 (2.21) b |
23.48 (3.89) b |
Larch |
54.35 (0.63) a |
0.86 (0.041) c |
0.13 (0.002) a |
40.08 (1.08) b |
19.11 (0.68) c |
29.24 (0.87) ab |
21.46 (0.94) b |
63.32 (3.49) b |
25.01 (2.04) b |
Birch |
49.69 (1.45) ab |
1.33 (0.022) a |
0.09 (0.004) c |
25.06 (1.96) d |
11.43 (0.75) d |
27.74 (0.94) b |
50.96 (0.96) a |
37.24 (1.35) c |
38.19 (1.01) a |
Willow |
45.23 (1.65) b |
1.15 (0.028) b |
0.11 (0.002) b |
41.71 (0.32) ab |
18.48 (1.57) c |
28.56 (1.88) b |
26.15 (3.29) b |
39.49 (2.18) c |
22.79 (2.45) b |
Azalea |
50.29 (1.60) a |
0.67 (0.020) d |
0.11 (0.009) bc |
43.14 (1.16) a |
25.84 (2.29) b |
27.00 (0.59) b |
21.84 (3.42) b |
75.54 (4.47) a |
32.90 (6.13) ab |
Methodology
This research was carried out at the Alpine Forest Ecosystems Long-Term Research Station. In 2009, three long-term sites with equivalent topographies and canopy covers were created in this alpine forest, resulting in related snowfall and litter fall. Each site had four plots: two in an open canopy to replicate full snow cover (control) and two in a confined canopy to simulate lowered snow cover (reduced snow cover).
The long-term decomposition of five dominant foliar fresh plant litters was investigated using an in situ litterbag technique. Samplings were taken based on the experimental procedures in other cold biomes and the temperature data in this alpine forest (Figure 1).
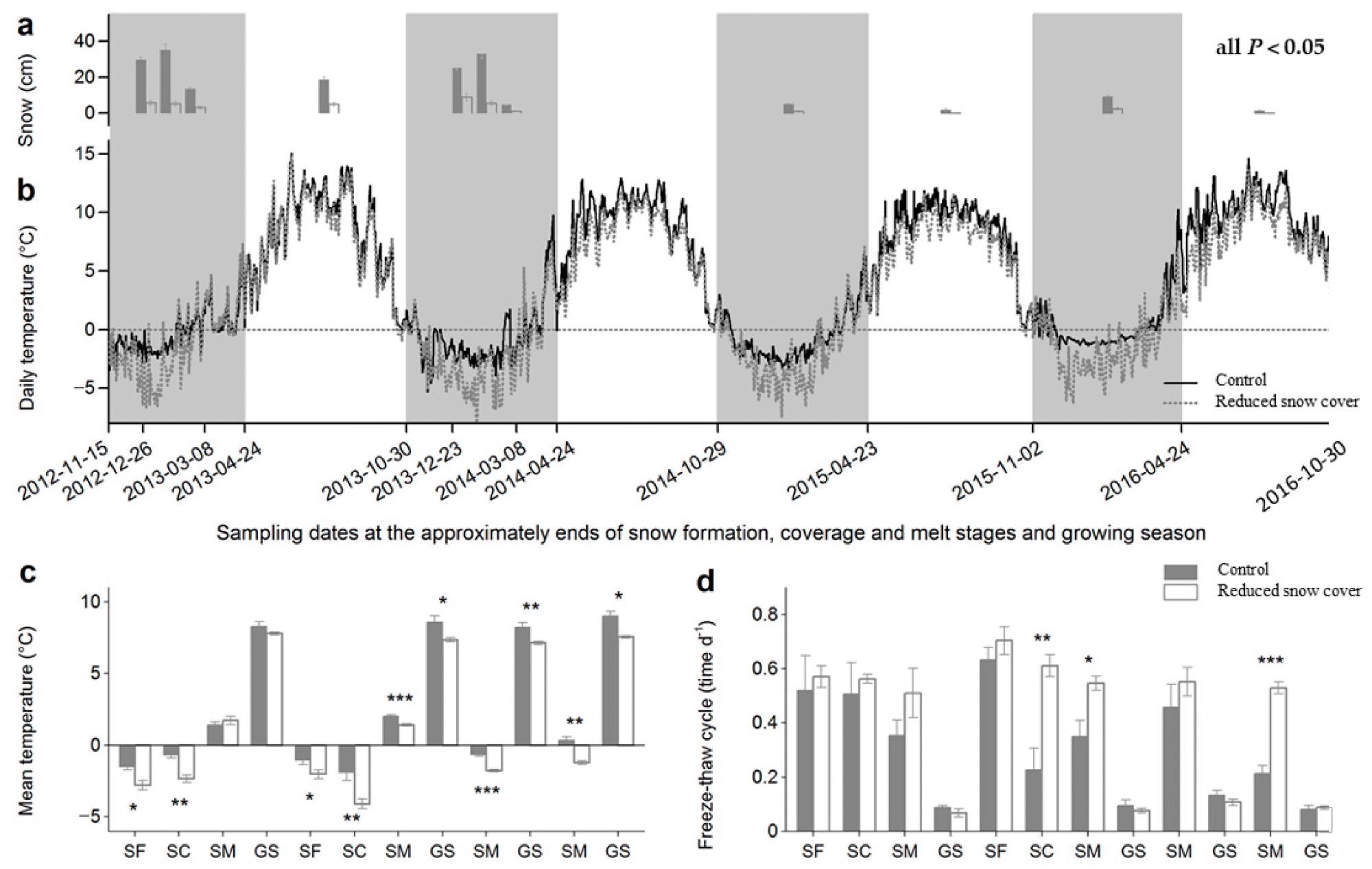
Figure 1. Temperatures and freeze–thaw cycles in the control and reduced snow cover plots. (a) Snow depths (±SE, n = 18) on each sampling date. Differences between control and reduced snow cover plots were significant (p < 0.05) on all sampling dates. (b) Daily temperatures (n = 6) at the litter surface during the four years of decomposition. Sampling dates were scheduled at approximately the ends of the snow formation, coverage and melt stages, and the growing season. Winter times, with the snow formation, coverage and melt stages, are shaded. The dashed line is drawn at a daily temperature of zero. (c) Mean temperatures (± SE, n = 6) at individual stages. Times between two adjacent sampling dates were defined as separate stages to better understand the effect of snow reduction at the snow formation, coverage and melt stages. SF: snow form stage, SC: snow cover stage, SM: snowmelt stage, GS: growing season. (d) Freeze–thaw cycles (±SE, n = 6) at the individual stages. Values were calculated using the quotients of the total number of freeze–thaw cycles and the days of certain stages. *p < 0.05, **p < 0.01, ***p < 0.001. Image Credit: Wang et al., 2022
To investigate the temporal influence of snow reduction on litter humification, samples were collected at the end of the snow formation, coverage, and melt stages in winter, as well as at the end of the growing season (beginning of snowfall). Figure 1 shows all of the sampling dates.
The samples were transported to the lab, cleaned, and their dry mass and water content were determined. In the foliar litter, another subsample was used to measure the overall humus, humic acid, and fulvic acid contents. The humus in the foliar litter was extracted using the alkali method. Humic and fulvic acids have been isolated.
Results
After four years of humification, the initial humus content (alkali-extractable substances) in the foliar litters accelerated to 16–21% across all species in the control plots (Figure 2).
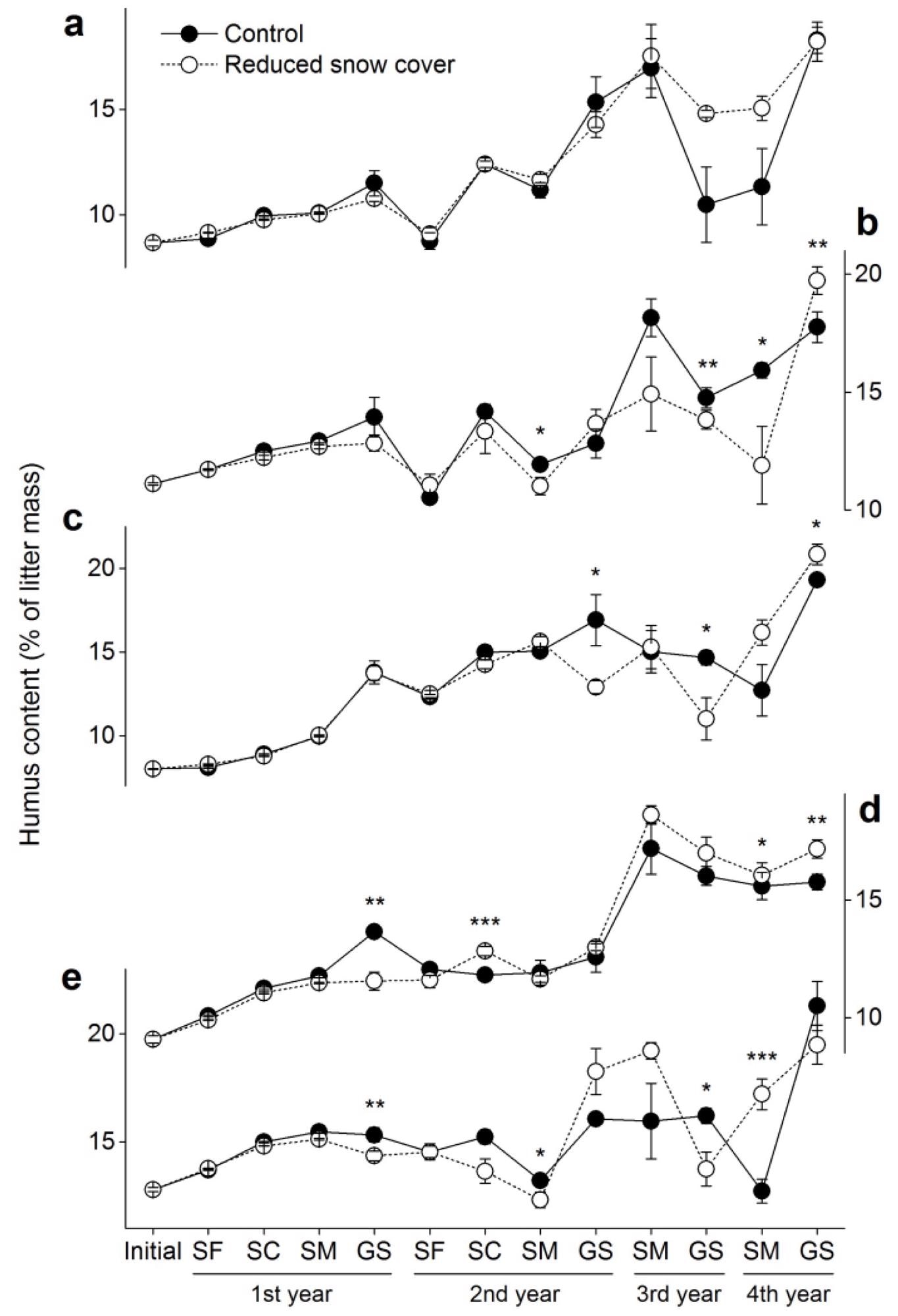
Figure 2. Humus contents (± SE, n = 6) in the five foliar litters. (a) Cypress, (b) larch, (c) birch, (d) willow and (e) azalea. SF: snow formation stage, SC: snow cover stage, SM: snowmelt stage, GS: growing season. * p < 0.05, ** p < 0.01, *** p < 0.001. Image Credit: Wang et al., 2022
The humus content remained stable but began to rise dramatically in the second growing season. Snow manipulation had no effect on humus content (Table 3), but it did have significant interactions with sampling time and litter species.
Table 3. Results of three-way ANOVA testing for the effects of time, litter species and snow manipulation. Bold values are significant (p < 0.05). Source: Wang et al., 2022
Source of Variation |
df |
Humus |
Humic Acid |
Fulvic Acid |
F Value |
p-Value |
F Value |
p-Value |
F Value |
p-Value |
Time |
11 |
112.0 |
<0.001 |
300.7 |
<0.001 |
63.1 |
<0.001 |
Litter |
4 |
59.6 |
<0.001 |
50.0 |
<0.001 |
22.6 |
<0.001 |
Snow |
1 |
0.02 |
0.90 |
6.0 |
0.015 |
2.1 |
0.14 |
Time × Litter |
44 |
7.5 |
<0.001 |
15.9 |
<0.001 |
6.4 |
<0.001 |
Time × Snow |
11 |
2.2 |
0.015 |
5.8 |
<0.001 |
3.1 |
<0.001 |
Litter × Snow |
4 |
2.9 |
0.022 |
2.0 |
0.097 |
5.3 |
<0.001 |
The plots with less snow cover had higher values at the end of the experiment. When all litter species were integrated, the humus content increased substantially (Figure 3) while the remaining litter mass decreased.
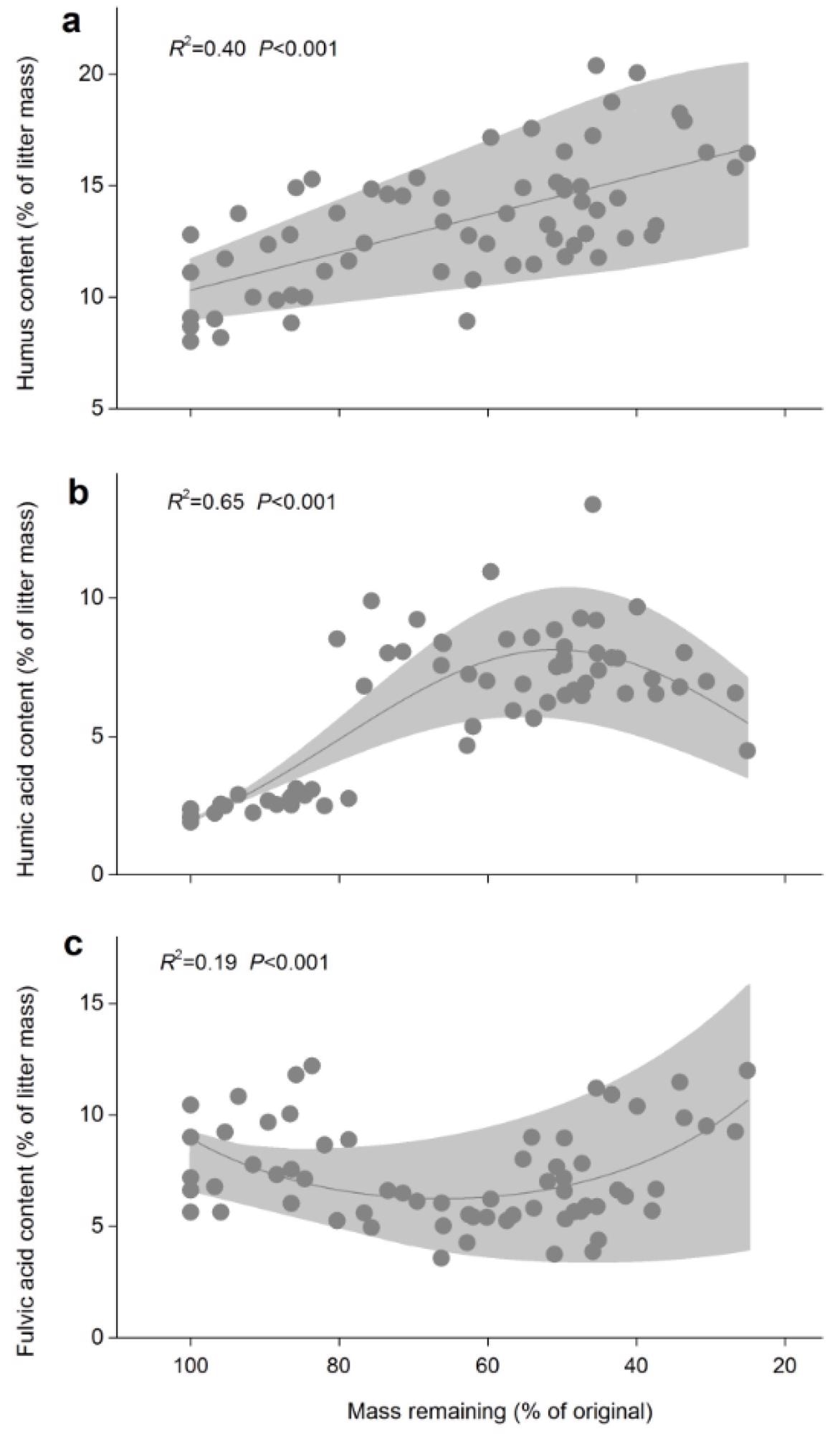
Figure 3. Relationships between the remaining mass and humus contents (a), humic acid contents (b) and fulvic acid contents (c). Shaded areas are 95% confidence intervals. Adjusted R2 and p-values from linear or exponential regressions are shown in each panel (all n = 78). Image Credit: Wang et al., 2022
The most important factor influencing humus content was manganese (Mn), and the impact of acid-unhydrolyzable residue (AUR) was larger than that of labile carbon, though neither was substantial and had VIP values below one (Figure 4).
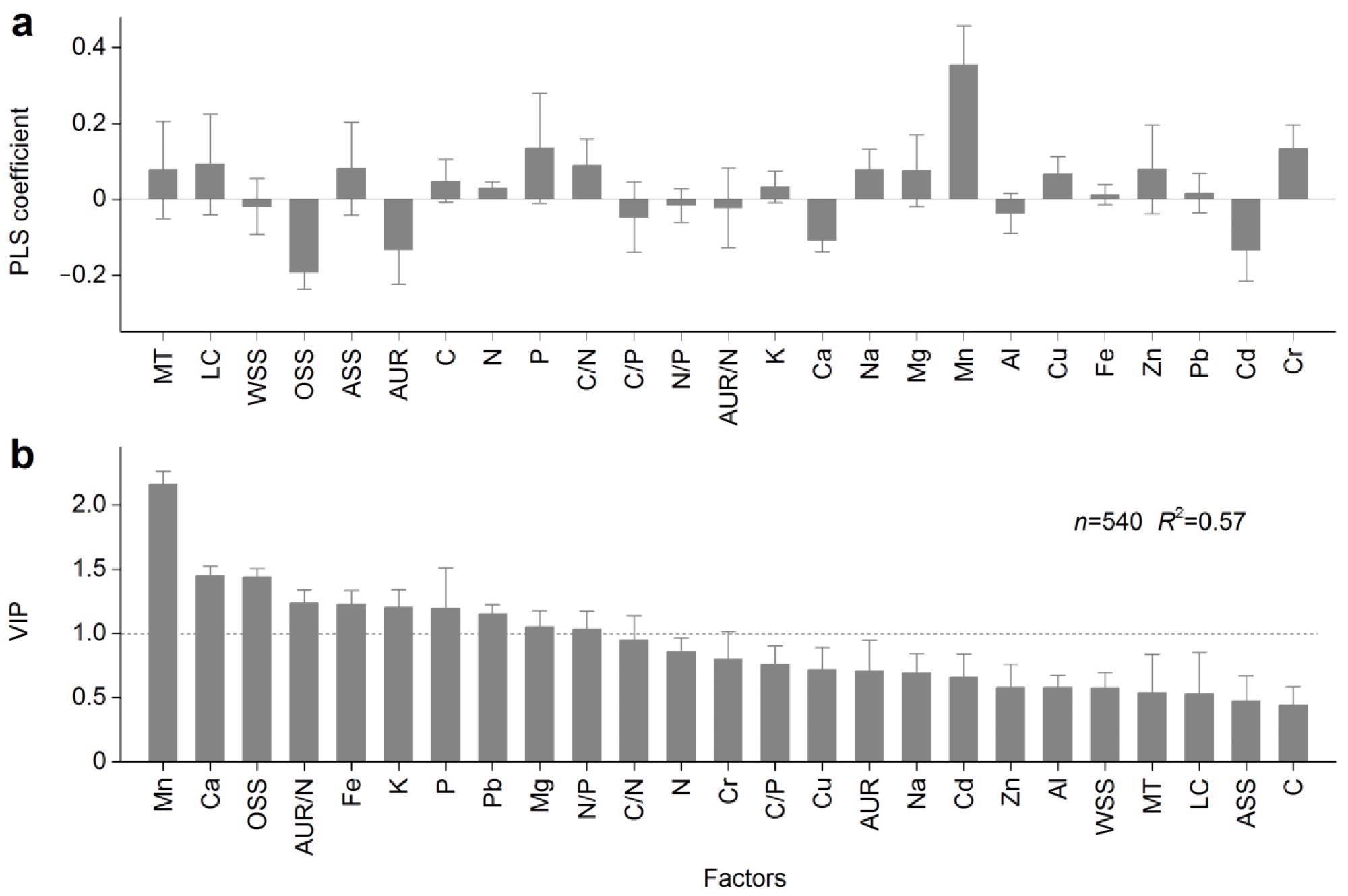
Figure 4. Partial least squares (PLS) analysis results for testing factors that impacted the humus content. (a) PLS coefficient. (b) Variable importance of projection (VIP). Error bars represent standard errors. VIP values greater than 1 (above the dashed line) were significant. MT: mean temperature, LC: labile carbon, WSS: water-soluble substances, OSS: organic-soluble substances, ASS: acid-soluble substances, AUR: acid-unhydrolyzable residue, C: carbon: N: nitrogen, P: phosphorus, K: potassium, Ca: calcium, Na: sodium, Mg: magnesium, Mn: manganese, Al: aluminum, Cu: copper, Fe: iron, Zn: zinc, Pb: lead, Cd: cadmium, Cr: chromium. All these factors were monitored in the long-term experiment. Image Credit: Wang et al., 2022
The initial humus content (alkali-extractable substances) in the foliar litter was 1.9–2.4%, and after four years of humification, it accelerated to 4.3–8.9% across all species in the control plots (Figure 5). The humic acid content remained stable throughout the first growing season, but it increased dramatically. The humic acid content was significantly affected by snow manipulation (Table 3).
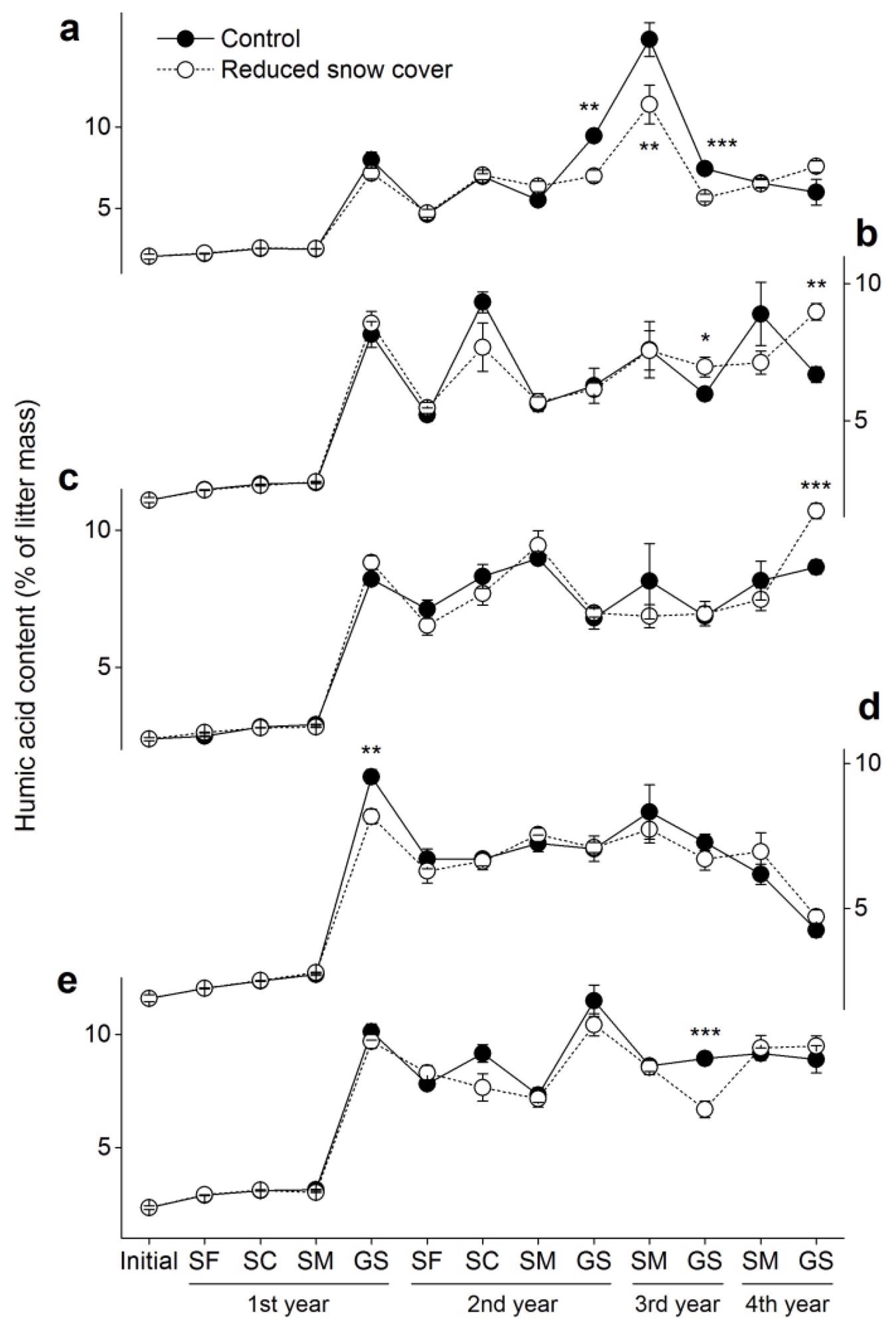
Figure 5. Humic acid contents (±SE, n = 6) in the five foliar litters. (a) Cypress, (b) larch, (c) birch, (d) willow and (e) azalea. SF: snow formation stage, SC: snow cover stage, SM: snowmelt stage, GS: growing season. * p < 0.05, ** p < 0.01, *** p < 0.001. Image Credit: Wang et al., 2022
The initial fulvic acid content of the foliar litter was 5.6–10.4%, but after four years of humification, it accelerated to 10.6–12.4% (Figure 6). During the first winter, the fulvic acid content was stable, but it dropped dramatically in the first growing season, then rebounded in the third year. The amount of fulvic acid in the snow did not change significantly.
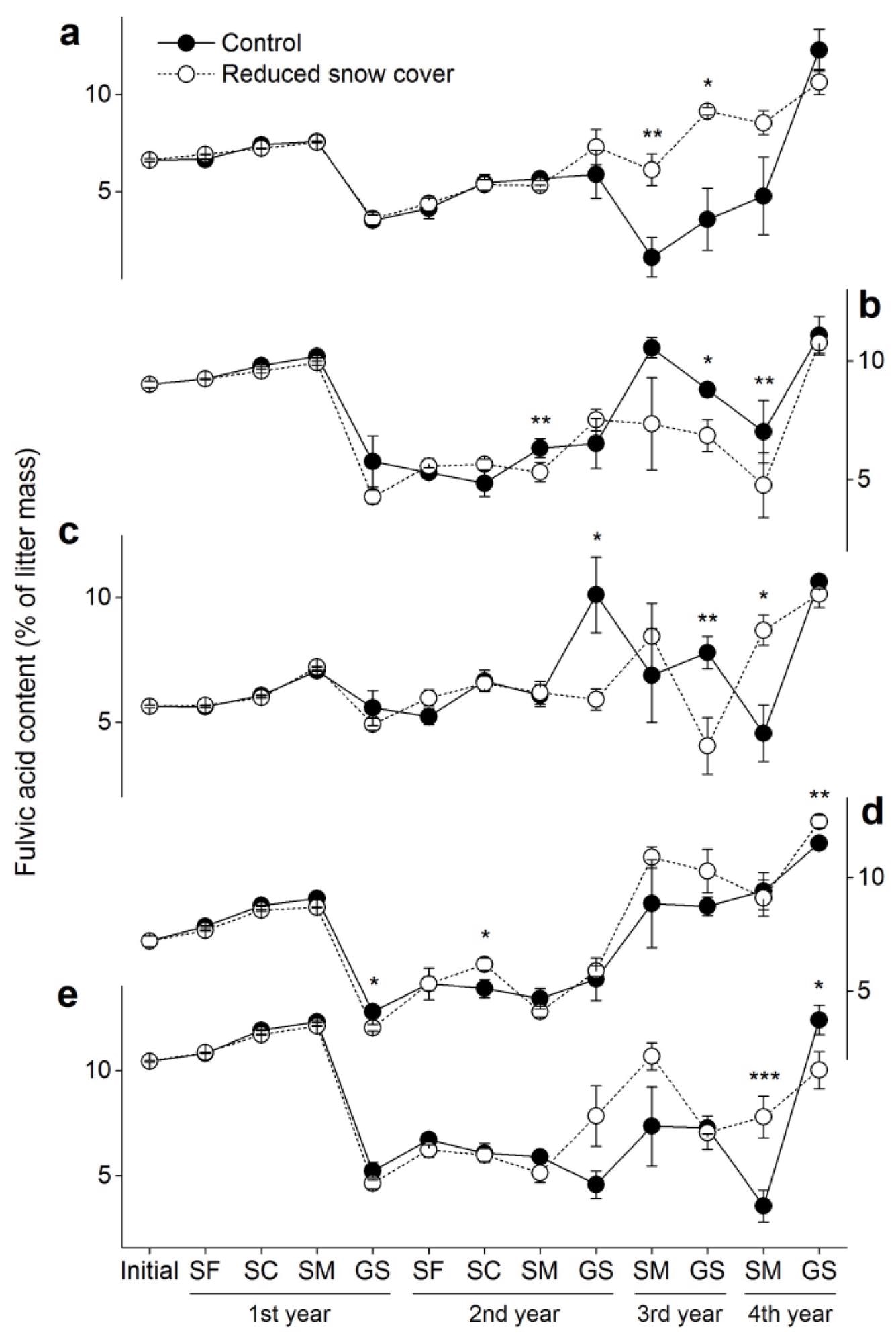
Figure 6. Fulvic acid contents (±SE, n = 6) in the five foliar litters. (a) Cypress, (b) larch, (c) birch, (d) willow and (e) azalea. SF: snow formation stage, SC: snow cover stage, SM: snowmelt stage, GS: growing season. * p < 0.05, ** p < 0.01, *** p < 0.001. Image Credit: Wang et al., 2022
Discussion
The initial humus content of the litter mass is attributed to 8–13% of the litter mass in this research (Figure 2), indicating a high degree of humification in freshly senesced foliage. Furthermore, as decomposition progressed (Figure 3a), the humus content steadily increased.
During the decomposition of litter, the researchers observed certain chemical compounds and mineral elements in this experiment. It was discovered that AUR had a stronger impact than labile carbon (Figure 4b). The findings also suggested that humus–mineral element bonding (for example, calcium and iron) may be more essential than chemical compounds in sustaining the organo-mineral stability of newly formed humus during the initial stages of litter decomposition.
In the decomposing foliar litter, fulvic acid forms before humic acid. However, at earlier stages of humification, the newly formed fulvic acid was unstable and degraded, whereas humic acid increased greatly before the remaining mass reached 50–60%. The findings highlighted the importance of conducting more long-term ecological research to address how carbon is sequestered in soils rather than how carbon is lost from plant litter.
Reduced snow cover lowered humus content in decomposing litter in the first three years, but increased greatly in the fourth year, according to this study (Figure 2). This finding suggests that short-term assessments may overestimate the true impact of reduced winter snow cover, which has a much more complicated effect on soil carbon sequestration, as earlier snow manipulation studies have shown.
Conclusion
After four years of humification, 16–21% of the residual plant litter was sequestered as stable humic substances in the substrate material, according to the in situ litterbag experiments. Despite the fact that this proportion was low compared to the decomposed amount (57–76%), carbon was maintained to some extent in decomposing litter throughout its early decay periods, when an organo-mineral association was shaped via mineral element connections with newly accumulated humus.
Journal Reference:
Wang, D., Ni, X., Guo, H., Dai, W. (2022) Alpine Litter Humification and Its Response to Reduced Snow Cover: Can More Carbon Be Sequestered in Soils? Forests, 13(6), p. 897. Available Online: https://www.mdpi.com/1999-4907/13/6/897/htm.
References and Further Reading
- Berg, B & McClaugherty, C (2020) Plant Litter: Decomposition, Humus Formation, Carbon Sequestration, 4th ed.; In: Springer: New York, NY, USA, pp. 13–43. Available at: https://link.springer.com/book/10.1007/978-3-030-59631-6?
- Bailey, V. L., et al. (2019) What do we know about soil carbon destabilization? Environmental Research Letters, 14(8), p. 083004. doi.org/10.1088/1748-9326/ab2c11.
- Tie, L., et al. (2022) The amounts and ratio of nitrogen and phosphorus addition drive the rate of litter decomposition in a subtropical forest. Science of The Total Environment, 833, p. 155163. doi.org/10.1016/j.scitotenv.2022.155163.
- Moore, C. J., et al. (2017) Astrometric search method for individually resolvable gravitational wave sources with Gaia. Physical Review Letters, 119(26), p. 261102. doi.org/10.1103/PhysRevLett.119.261102.
- Prescott, C E (2010) Litter decomposition: What controls it and how can we alter it to sequester more carbon in forest soils? Biogeochemistry, 101, pp. 133–149. doi.org/10.1007/s10533-010-9439-0.
- Schmidt, M., et al. (2011) Persistence of soil organic matter as an ecosystem property. Nature, 478, pp. 49–56. doi.org/10.1038/nature10386.
- Lehmann, J & Kleber, M (2015) The contentious nature of soil organic matter. Nature, 528, pp. 60–68. doi.org/10.1038/nature16069.
- Rubino, M., et al. (2010) Carbon input belowground is the major C flux contributing to leaf litter mass loss: Evidences from a C-13 labelled-leaf litter experiment. Soil Biology and Biochemistry, 42, pp. 1009–1016. doi.org/10.1016/j.soilbio.2010.02.018.
- Haddix, M. L., et al. (2016) Dual, differential isotope labeling shows the preferential movement of labile plant constituents into mineral-bonded soil organic matter. Global Change Biology, 22(6), pp. 2301–2312. doi.org/10.1111/gcb.13237.
- Olk, D. C., et al. (2019) Environmental and agricultural relevance of humic fractions extracted by alkali from soils and natural waters. Journal of Environmental Quality, 48(2), pp. 217–232. doi.org/10.2134/jeq2019.02.0041.
- Cotrufo, M. F., et al. (2015) Formation of soil organic matter via biochemical and physical pathways of litter mass loss. Nature Geoscience, 8, pp. 776–779. doi.org/10.1038/ngeo2520.
- Pries, C. E. H., et al. (2017) Long term decomposition: The influence of litter type and soil horizon on retention of plant carbon and nitrogen in soils. Biogeochemistry, 134, pp. 5–16. doi.org/10.1007/s10533-017-0345-6.
- Cotrufo, M. F., et al. (2013) The Microbial Efficiency-Matrix Stabilization (MEMS) framework integrates plant litter decomposition with soil organic matter stabilization: Do labile plant inputs form stable soil organic matter? Global Change Biology, 19(4), pp. 988–995. doi.org/10.1111/gcb.12113.
- Luojus, K., et al. (2018) Assessment of seasonal snow cover mass in northern hemisohere during the satellite-era. In: IGARSS 2018 - 2018 IEEE International Geoscience and Remote Sensing Symposium, Valencia, Spain, 22–27 July 2018. doi.org/10.1109/IGARSS.2018.8517494.
- Li, Z., et al. (2017) Effects of snow absence on winter soil nitrogen dynamics in a subalpine spruce forest of southwestern China. Geoderma, 307, pp. 107–113. doi.org/10.1016/j.geoderma.2017.08.003.
- Cooper, E J (2014) Warmer Shorter Winters Disrupt Arctic Terrestrial Ecosystems. Annual Review of Ecology, Evolution, and Systematics; 45, pp. 271–295. doi.org/10.1146/annurev-ecolsys-120213-091620.
- Marco-Barba, J., et al. (2013) The influence of climate and sea-level change on the Holocene evolution of a Mediterranean coastal lagoon: Evidence from ostracod palaeoecology and geochemistry. Geobios, 46, pp. 409–421. doi.org/10.1016/j.geobios.2013.05.003.
- Kreyling, J., et al. (2013) Erratum to: Absence of snow cover reduces understory plant cover and alters plant community composition in boreal forests. Oecologia, 173, p. 1157. doi.org/10.1007/s00442-013-2657-0.
- Saccone, P., et al. (2013) The effects of snowpack properties and plant strategies on litter decomposition during winter in subalpine meadows. Plant and Soil, 363, pp. 215–229. doi.org/10.1007/s11104-012-1307-3.
- Bastida, F., et al. (2021) Soil microbial diversity-biomass relationships are driven by soil carbon content across global biomes. The ISME Journal, 15, pp. 2081–2091. doi.org/10.1038/s41396-021-00906-0.
- Gavazov, K S (2010) Dynamics of alpine plant litter decomposition in a changing climate. Plant and Soil, 337, pp. 19–32. doi.org/10.1007/s11104-010-0477-0.
- Ni, X. Y., et al. (2014) The responses of early foliar litter humification to reduced snow cover during winter in an alpine forest. Canadian Journal of Soil Science, 94(4), pp. 453–461. doi.org/10.4141/cjss2013-121.
- Bradford, M. A., et al. (2013) Empirical evidence that soil carbon formation from plant inputs is positively related to microbial growth. Biogeochemistry, 113, pp. 271–281. doi.org/10.1007/s10533-012-9822-0.
- Xiang, B., et al. (2022) Influences of freezing-thawing actions on mechanical properties of soils and stress and deformation of soil slope in cold regions. Scientific Reports, 12, p. 5387. doi.org/10.1038/s41598-022-09379-3.
- Aanderud, Z. T., et al. (2013) Sensitivity of soil respiration and microbial communities to altered snowfall. Soil Biology and Biochemistry, 57, pp. 217–227. doi.org/10.1016/j.soilbio.2012.07.022.
- Hui, R., et al. (2019) Variation in snow cover drives differences in soil properties and microbial biomass of BSCs in the Gurbantunggut Desert-3 years of snow manipulations. Ecohydrology, 12(6), p. e2118. doi.org/10.1002/eco.2118.
- Castellano, M. J., et al. (2015) Integrating plant litter quality, soil organic matter stabilization, and the carbon saturation concept. Global Change Biology, 21(9), pp. 3200–3209. doi.org/10.1111/gcb.12982.
- Aerts, R., et al. (2012) Seasonal climate manipulations have only minor effects on litter decomposition rates and N dynamics but strong effects on litter P dynamics of sub-arctic bog species. Oecologia, 170, pp. 809–819. doi.org/10.1007/s00442-012-2330-z.
- Li, H., et al. (2016) Effects of forest gaps on litter lignin and cellulose dynamics vary seasonally in an alpine forest. Forests, 7(2), p. 27. doi.org/10.3390/f7020027.
- He, J., et al. (2016) Copper and zinc dynamics in foliar litter during decomposition from gap center to closed canopy in an alpine forest. Scandinavian Journal of Forest Research, 31(4), pp. 355–367. doi.org/10.1080/02827581.2015.1078405.
- Ni, X. Y., et al. (2015) Accelerated foliar litter humification in forest gaps: Dual feedbacks of carbon sequestration during winter and the growing season in an alpine forest. Geoderma,241–242, pp. 136–144. doi.org/10.1016/j.geoderma.2014.11.018.
- Zhao, Y., et al. (2016) Bacterial community changes during fir needle litter decomposition in an alpine forest in eastern Tibetan Plateau. Russian Journal of Ecology, 47, pp. 145–157. doi.org/10.1134/S1067413616020156.
- Wu, F., et al. (2014) Impacts of freezing and thawing dynamics on foliar litter carbon release in alpine/subalpine forests along an altitudinal gradient in the eastern Tibetan Plateau. Biogeosciences, 11, p. 6871. doi.org/10.5194/bg-11-6871-2014.
- Wu, F. Z., et al. (2010) Litter decomposition in two subalpine forests during the freeze-thaw season. Acta Oecologica, 36(1), pp. 135–140. doi.org/10.1016/j.actao.2009.11.002.
- Ladwig, L. M., et al. (2016) Beyond arctic and alpine: The influence of winter climate on temperate ecosystems. Ecology, 97(2), pp. 372–382. doi.org/10.1890/15-0153.1.
- Ni, X. Y., et al. (2016) Forest gaps slow the sequestration of soil organic matter: A humification experiment with six foliar litters in an alpine forest. Scientific Reports, 6, p. 19744. doi.org/10.1038/srep19744.
- Konestabo, H. S., et al. (2007) Responses of springtail and mite populations to prolonged periods of soil freeze-thaw cycles in a sub-arctic ecosystem. Applied Soil Ecology, 36(2–3), pp. 136–146. doi.org/10.1016/j.apsoil.2007.01.003.
- Lan, L. Y., et al. (2019) Effects of soil fauna on microbial community during litter decomposition of Populus simonii and Fargesia spathacea in the subalpine forest of western Sichuan, China. The Journal of Applied Ecology, 30(9), pp. 2983–2991. doi.org/10.13287/j.1001-9332.201909.033.
- Mehmood, T., et al. (2012) A review of variable selection methods in Partial Least Squares Regression. Chemometrics and Intelligent Laboratory Systems, 118, pp. 62–69. doi.org/10.1016/j.chemolab.2012.07.010.
- Trap, J., et al. (2017) Slow decomposition of leaf litter from mature Fagus sylvatica trees promotes offspring nitrogen acquisition by interacting with ectomycorrhizal fungi. Journal of Ecology, 105(2), pp. 528–539. doi.org/10.1111/1365-2745.12665.
- Oldfield, E. E., et al. (2018) Substrate identity and amount overwhelm temperature effects on soil carbon formation. Soil Biology and Biochemistry, 124, pp. 218–226. doi.org/10.1016/j.soilbio.2018.06.014.
- Hall, S. J., et al. (2020) Lignin lags, leads, or limits the decomposition of litter and soil organic carbon. Ecology, 101(9), p. e03113. doi.org/10.1002/ecy.3113.
- Berg, B., et al. (2021) Magnesium dynamics in decomposing foliar litter-A synthesis. Geoderma, 382, p. 114756. doi.org/10.1016/j.geoderma.2020.114756.
- Berg, B., et al. (2015) Manganese in the litter fall-forest floor continuum of boreal and temperate pine and spruce forest ecosystems-A review. Forest Ecology and Management, 358, pp. 248–260. doi.org/10.1016/j.foreco.2015.09.021.
- Qualls, R. G., et al. (2003) Formation and loss of humic substances during decomposition in a pine forest floor. Soil Science Society of America Journal, 67(3), pp. 899–909. doi.org/10.2136/sssaj2003.8990.
- Christenson, L. M., et al. (2010) Winter climate change implications for decomposition in northeastern forests: Comparisons of sugar maple litter with herbivore fecal inputs. Global Change Biology, 16(9), pp. 2589–2601. doi.org/10.1111/j.1365-2486.2009.02115.x.
- Blok, D., et al. (2016) Initial stages of tundra shrub litter decomposition may be accelerated by deeper winter snow but slowed down by spring warming. Ecosystems, 19, pp. 155–169. doi.org/10.1007/s10021-015-9924-3.
- Baptist, F., et al. (2010) Direct and indirect control by snow cover over decomposition in alpine tundra along a snowmelt gradient. Plant and Soil, 328, pp. 397–410. doi.org/10.1007/s11104-009-0119-6.
- Bokhorst, S., et al. (2010) Impacts of extreme winter warming events on litter decomposition in a sub-Arctic heathland. Soil Biology and Biochemistry, 42, pp. 611–617. doi.org/10.1016/j.soilbio.2009.12.011.
- Bokhorst, S., et al. (2013) Reduction in snow depth negatively affects decomposers but impact on decomposition rates is substrate dependent. Soil Biology and Biochemistry, 62, pp. 157–164. doi.org/10.1016/j.soilbio.2013.03.016.
- Dungait, J. A. J., et al. (2012) Soil organic matter turnover is governed by accessibility not recalcitrance. Global Change Biology, 18(6), pp. 1781–1796. doi.org/10.1111/j.1365-2486.2012.02665.x.