AZoCleantech speaks to Dr Andrea (Annie) Kritcher of the National Ignition Facility about the team's important fusion breakthrough that could open up the possibility of limitless, clean energy. The US team yielded a record-breaking burst of energy of more than 10 quadrillion watts using a ground-breaking method of creating nuclear fusion.
What kind of research is carried out at the National Ignition Facility and what technology is currently being used?
NIF is a research facility that provides access to key physics regimes which are only otherwise accessible through nuclear explosive testing or astrophysical plasmas. NIF conducts experiments to support the U.S. Stockpile Stewardship Program, including fusion ignition experiments, and provides fundamental understanding for astrophysics and high energy density plasmas, for example. The recent experiments described here demonstrate that NIF can provide access to the physics regime around ignition, which creates energy through fusion reactions in a robust energy feedback process from self-heating of the plasma.
The technology at NIF is to shine intense laser beams onto sample materials about the size of a pencil eraser to create extreme states of matter which replicate those found in the center of the sun, giant planets, and white dwarf stars. In recent experiments, a little over 1.9 million Joules was delivered to the target in 9 billionths of a second with maximum laser power of 440 trillion watts.
The lasers create a radiation field inside the target that is used to implode a capsule containing heavy isotopes of hydrogen. The implosion process results in extreme densities and temperatures inside the fuel, which causes the hydrogen isotopes to fuse together and release high-energy neutrons and helium particles. This process requires extreme temperatures of ~100 million degrees and has recently produced 10 quadrillion watts of power, but for a fraction (90 trillionths) of a second.
How did you begin your research into fusion ignition?
I joined the lab as a summer student in 2004 in the area of nuclear physics while attending the University of Michigan. I was immediately drawn to “the big laser project” and intrigued by the idea of a grand scientific challenge. Since opportunities to be a part of something like this do not come around every career, I knew I had to join.
I attended the University of California Berkeley to be close to the lab during my graduate and postdoc work with Siegfried Glenzer as an experimentalist, then joined the design program after my postdoc to design ignition experiments with Omar Hurricane and Debbie Callahan. I am very grateful for having the opportunity to be the lead designer on these experiments and be a part of something this big in my career.
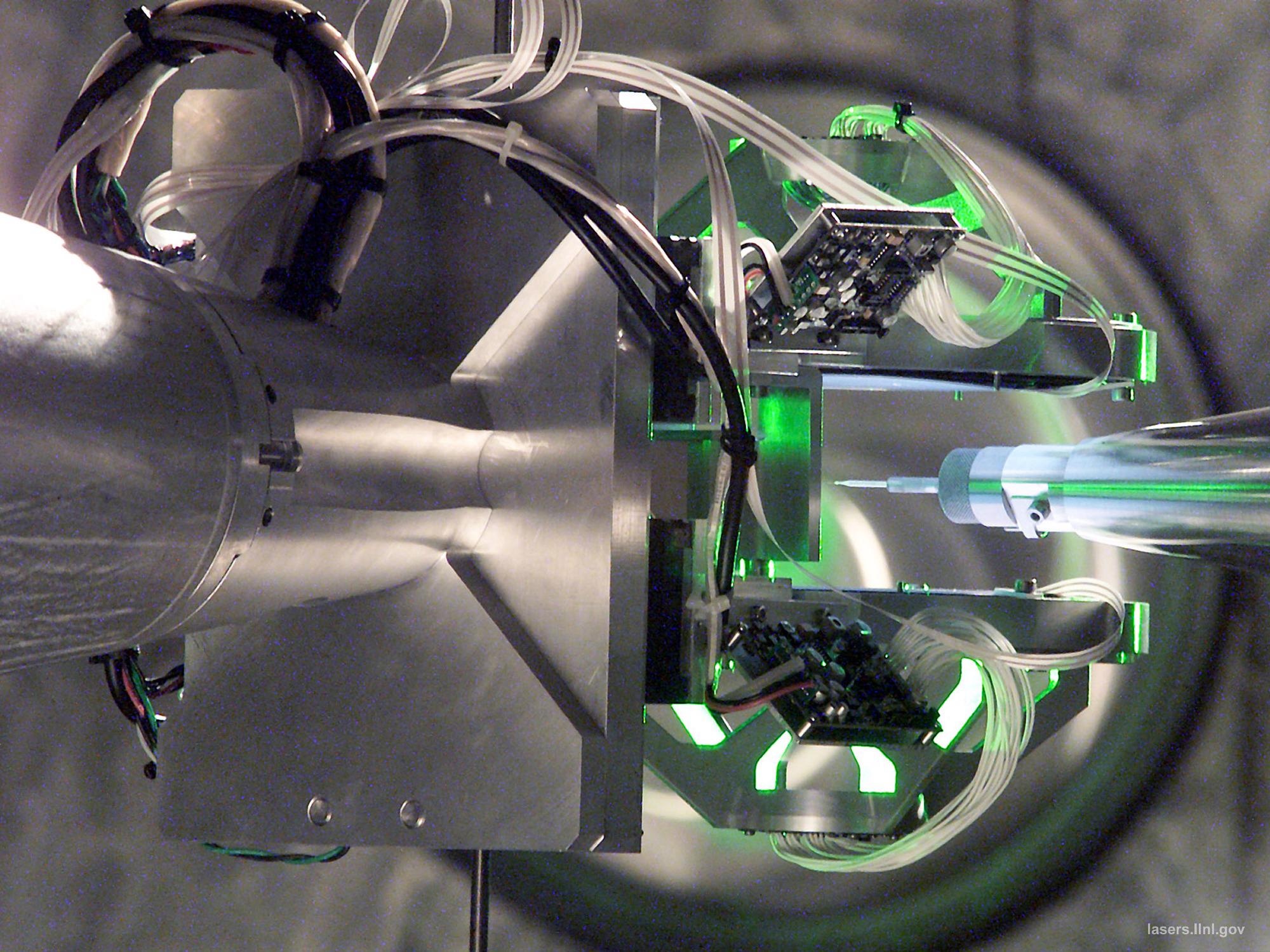
Dr Andrea Kritcher and her team have offered the first demonstration of self-sustaining nuclear fusion in the laboratory. Image Credit: Lawrence Livermore National Laboratory
Please can you explain the experiment your team conducted and what you set out to achieve?
The team has been developing a platform to increase implosion size beyond what was explored in 2017 and 2018 while maintaining many other implosion properties required to heat and compress the fuel efficiently.
The team's most recent experiment was to take the previous highest fusion energy output experiment from this campaign and further increase the heating and compression of the central fuel through improvements to the radiation drive created by the lasers. We expected to see the fuel temperature increase and the number of fusion reactions increase as a result.
What key findings came out of the experiment?
The result was an 8X improvement over experiments conducted in spring 2021 and a 25X increase over NIF’s 2018 record yield. For the first time on any fusion research facility, we have output 70% of the laser energy delivered to the target as fusion energy and exceeded the energy delivered to the capsule that contains the fuel by more than five times. This milestone has been sought after for decades by many people. Creating these conditions in the laboratory will significantly advance our understanding of what it takes to achieve energy gain higher than required to create the fusion, and provides access to a new experimental regime that was previously unattainable.
The National Ignition Facility experiment is close to achieving a key nuclear fusion goal. What would this mean for clean energy? What are the current limitations in nuclear energy and how could this research prove vital in revolutionizing its future?
Reaching a fusion energy gain of 100% of the incident laser energy will mean that we have generated more fusion energy than the laser energy required to create the fusion reactions. While reaching this goal is important and what was defined as a milestone in NIFs beginnings, our result (fusion energy that is 70% of the incident laser energy) is already of great scientific importance for fusion energy research.
This research is the first demonstration of self-sustaining nuclear fusion in the laboratory, which occurs when the energy being emitted by the fusion outweighs energy loss mechanisms and external energy needed to create the fusion is no longer needed. This is an essential first demonstration for future clean energy facilities which aim to generate more energy than required to initiate the fusion reactions.
A previous skeptic of fusion ignition on NIF iterates the importance of this work for fusion energy by stating “It demonstrates to the skeptic that there is nothing fundamentally wrong with the laser fusion concept,” [Steve Bodner] said. “It is time for the U.S. to move ahead with a major laser fusion energy program.”
The recent findings are just the beginning and much more work is needed to make this a practical application for fusion energy. NIF is a research facility and was not designed to conduct these experiments at a rate that would support energy delivery. Fusion ignition is only one part of a future fusion energy program and it will be up to our sponsors and Congress to determine the next steps on the path to fusion energy delivery.
What is inertial confinement fusion and how does this process kickstart thermonuclear fusion?
Inertial confinement fusion is a method for creating nuclear fusion through heating and compressing heavy isotopes of hydrogen (fuel) to extreme temperatures and densities. The NIF laser beams are used to heat the outside of a pellet that contains the fuel, which is about the size of a pinhead. This heating causes the fuel to be accelerated inward, compressing the fuel to conditions where fusion reactions can occur.
The indirect drive approach uses a gold or depleted uranium can the size of a pencil eraser to convert the laser energy to a radiation drive that heats the outside of the fuel pellet. The inertial confinement fusion approach at NIF creates controlled thermonuclear burn.
Can you explain what ‘burning plasma’ is and how this process was achieved?
A burning plasma refers to a plasma state where heating from alpha particle deposition (heating by the alpha particles created by the fusion reactions) is the dominant heating source in the plasma. More specifically, when the plasma's self-heating is greater than the energy imparted on the plasma and the energy loss terms.
These experiments have reached conditions where the fusion energy created is >5 times the energy imparted to the pellet due to robust feedback from the alpha particle self-heating.
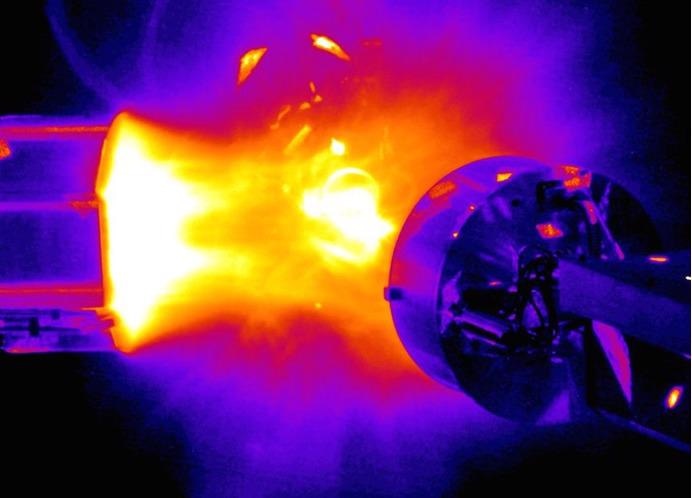
Shot-time image from the NIF Target Chamber of an x-ray source creating a plasma wind on system-generated electromagnetic pulse (SGEMP) test objects. Image Credit: Lawrence Livermore National Laboratory
Have you faced any challenges during your research and how were or will these be overcome?
To create the extreme conditions needed to achieve ignition, these experiments require a great deal of precision in many aspects of the experiment, including laser delivery, target fabrication, and control of the symmetry of the imploding pellet and hydrodynamic instabilities. Many aspects need to come into place for the best experimental conditions, regardless of the intended design.
Developments by many people who support these experiments have made this possible, including improvements to the engineering features and laser capability. In addition, modeling these extreme plasma conditions is very difficult. Knowing where the models can be trusted and where semi-analytical models were needed to make progress was important in this research. Design techniques for improving implosion efficiency through minimizing hydrodynamic instabilities and maintaining symmetry while considering the experimental constraints and balancing design optimization was important. This has been a continuous learning process and has enabled recent progress.
What are the next steps in this research?
Future experiments aim to understand the new physics regime we have recently accessed through advanced diagnostics and assessing performance reproducibility. Future work also includes continuing to improve on the recent results by increasing the compression of the fuel and improve implosion efficiency by minimizing perturbations and continuing to develop the radiation drive.
Where can readers find more information?
This experiment builds on and extends experiments in the spring, some of which are summarized in a Physics of Plasmas paper that can be found at https://aip.scitation.org/doi/full/10.1063/5.0047841 and https://journals.aps.org/prl/abstract/10.1103/PhysRevLett.126.025001. The most recent experiments are being submitted for publication.
About Dr. Annie Kritcher
Dr. Annie Kritcher is the design lead within the ICF program for campaigns to increase implosion scale on the NIF and is a member of the ICF leadership team. She serves as team lead for integrated implosion modeling which oversees all experiments to increase energy coupling on the NIF. She is also a group leader within the design physics division at LLNL. Her main responsibilities as a campaign lead include setting the strategic direction of the campaign with an experimental co-lead, leading the design effort and post-shot analysis, interpretation of data, collaboration with other campaigns, and interfacing with target fabrication. Annie has served as the chair of the Strategic Initiative and Exploratory Research High Energy Density Science LDRD technical review committee, the Lawrence Fellow selection committee, Dynamic Compression Sector proposal selection committee, and the LCLS MEC peer review panel.
Annie was first employed at the Lab as a summer intern in 2004, as an LLNL Lawrence Scholar during her time at UC Berkeley, and as a Lawrence postdoctoral fellow in 2009 following completion of her Ph.D. Annie’s thesis on experimental measurements of X-ray Thomson Scattering to diagnose high energy density matter was conducted at the Jupiter laser facility (LLNL) and Omega laser facility (Rochester) which is featured in Science.
During her postdoctoral appointment, she continued her work on X-ray Thomson Scattering, investigated nuclear plasma interactions, and co-lead a campaign to measure the equation of state of materials to hundreds of Mbars which is featured in Nature. She also obtained an LDRD to continue this project after her postdoc. Following her postdoc, she transitioned from experimental physics to design physics within the Design Physics directorate at LLNL where her main focuses included assessing the impact of low mode asymmetries on ICF implosions, ICF ablator material comparison, and increasing ICF implosion scale. This work has led to the highest fusion yields ever achieved in the laboratory (>1.35 MJ) and will be submitted to Nature.
Disclaimer: The views expressed here are those of the interviewee and do not necessarily represent the views of AZoM.com Limited (T/A) AZoNetwork, the owner and operator of this website. This disclaimer forms part of the Terms and Conditions of use of this website.