Recent developments in fusion energy modeling, such as a new “nonperturbative guiding center model,” enhance scientists’ ability to simulate plasma behavior in fusion reactors. These improvements address long-standing challenges to make fusion energy more practical.
Fusion, the process powering the sun, is explored as a clean energy source, but developing it into a reliable power solution requires overcoming complex scientific and engineering hurdles. This article looks at the basics of fusion energy, its benefits and challenges, and the ongoing research progress toward making it a viable part of our energy future.
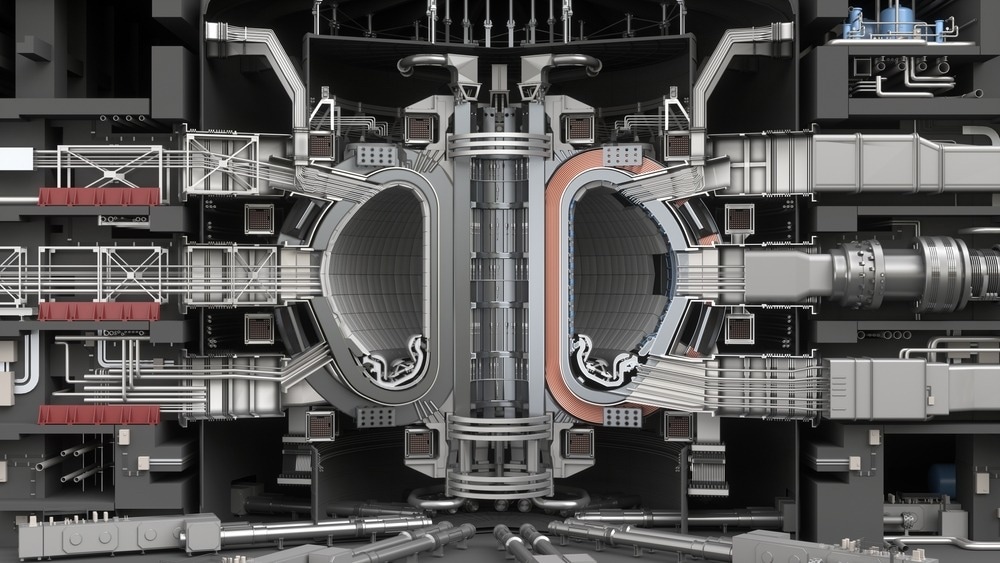
Image Credit: Borshch Filipp/Shutterstock.com
What is Fusion Energy?
Fusion energy mimics the sun, where light atomic nuclei (such as hydrogen) merge at extremely high temperatures and pressures, forming heavier elements and releasing energy. In most experiments, this process uses hydrogen isotopes such as deuterium and tritium as fuel. One of fusion’s main appeals is the potential availability of its fuel. Deuterium, for instance, can be extracted from seawater, offering a relatively accessible and long-lasting supply. One gram of deuterium-tritium fuel could produce as much energy as approximately 20 tons of coal, highlighting the high energy density of fusion reactions.1
Environmental Benefits of Fusion Energy
Fusion does not emit direct carbon, making it attractive for climate change mitigation.2 Compared to nuclear fission, fusion produces radioactive waste with a much shorter half-life, typically becoming safer within around 100 years, unlike fission waste that remains hazardous for thousands of years.1
Safety Advantages of Fusion Reactors
Fusion reactors cannot undergo a meltdown because the fusion reaction stops automatically if the extreme conditions (temperature, pressure, and magnetic fields) are not maintained.1 Unlike nuclear fission, fusion does not involve chain reactions, reducing the risk of large-scale accidents.3
Challenges in Making Fusion Energy Practical
Key challenges include sustaining extreme plasma temperatures and pressures, developing durable materials, and managing tritium fuel supply.
Plasma maintenance
Fusion requires temperatures over 100 million degrees Celsius to maintain plasma—ionized gas where electrons are separated from nuclei.4 Plasma is naturally unstable, and any minor disturbance can halt the reaction, making it challenging to sustain controlled fusion. 5
Material durability
Fusion reactors must withstand extreme heat, radiation from high-energy particles (called neutrons), and tritium exposure. This combined effect, called the “triple whammy”, causes materials to degrade over time.5, 6 Developing radiation-resistant materials is critical for reactor longevity.
Tritium fuel supply
Tritium is naturally rare and must be bred within reactors, complicating design and fuel cycle management. Efficient tritium handling is vital for safe, continuous fusion.4, 7
Progress in these areas is necessary for moving fusion energy from experimental stages toward becoming a reliable and practical power source.
Key Developments in Fusion Energy Research
Fusion energy research has progressed through two main approaches: magnetic confinement and inertial confinement. Magnetic confinement, especially using tokamaks, leads the field.
Tokamaks confine plasma with strong magnetic fields inside a doughnut-shaped chamber. A major example is the ITER project, an international effort to demonstrate a net energy gain by generating 500 MW of fusion power from a 50 MW input. However, superconducting magnets are costly, and issues like managing magnet quenches remain.8,9
To download this article, please click here
Stellarators provide an alternative to tokamaks using complex magnetic fields without internal electric currents. One of the leading projects in this area is the Wendelstein 7-X in Germany, which has improved magnetic configurations, enhancing plasma stability and runtime. However, despite progress, stellarators are complex and face engineering challenges.10, 11
In 2025, China’s EAST tokamak sustained plasma at 108 million °C for 1,066 seconds using lithium-treated walls and advanced current drive. The experiment operated in a high-confinement state called Super I-mode, which helped control heat and impurities effectively. 12
In inertial confinement fusion, the National Ignition Facility achieved a breakthrough in 2022 by producing more energy from fusion reactions than the energy put in, marking progress for laser-driven fusion technology. This development lays the foundation for further exploration of laser inertial fusion as a viable clean energy source, potentially enhancing energy security.13
Germany's New Nuclear Fusion Breakthrough SHOCKS The Entire Industry!
Video Credit: Quanta/YouTube.com
Latest Developments in Fusion Plasma Simulation
A recent breakthrough in fusion energy modeling is the "nonperturbative guiding center model" for magnetized plasmas, published in Physical Review Letters (2025). This new simulation approach helps scientists accurately predict the motion of charged particles in magnetic fusion devices.
Traditionally, simulations rely on approximations that can struggle with fast-moving, high-energy particles. This new model instead uses full-orbit simulations, tracking particles' paths precisely, even for alpha particles (helium nuclei created in fusion reactions) that play a vital role in maintaining the plasma’s heat.
Enhanced Computational Efficiency for Reactor Design
According to a recent study, the new model speeds up simulation time tenfold without sacrificing accuracy.15 This is especially helpful for designing stellarators, which involve testing many complex magnetic configurations.
It also helps researchers simulate runaway electrons, particles that gain dangerously high energy during plasma disruptions. If uncontrolled, these electrons can damage reactor walls, so predicting their behavior is critical for safe reactor operation.
Implications for Fusion Technology
Improved modeling helps address several practical challenges. The simulations also help identify where particles may interact with reactor walls, informing material choice and component design.
Increased computational efficiency allows the exploration of more design options, potentially enhancing reactor performance. While this work represents a significant technical advancement, the model requires further testing and integration into current design tools. Future work should extend it to full 3D reactor geometries and incorporate additional physics for comprehensive reactor analysis.
Remaining Challenges in Achieving Fusion Energy
Despite progress, several significant challenges remain before fusion energy can become a practical power source.
Maintaining stable plasma at over 100 million degrees Celsius without disruption remains difficult.4,5 Materials must endure combined damage from neutron bombardment, heat, and tritium exposure for long reactor lifetimes.5,6
Creating a self-sustaining tritium cycle remains complex and crucial. Addressing these physics and engineering challenges is essential for the future success of fusion power plants.
Is there a Future for Fusion Power?
Advancing fusion will require ongoing research on multiple fronts. Testing the new guiding center model against experimental data and incorporating it into comprehensive design software will enhance reactor development.
Expanding simulations to cover full 3D geometries and physical effects is also needed. Materials science research must identify substances that can survive reactor conditions long-term. Progress in tritium breeding and fuel cycle management is essential for steady operation.
Fusion energy is currently in a phase of gradual progress, where scientific understanding is growing, but practical implementation remains complex.
Although improvements in simulation models and reactor performance are essential steps forward, achieving reliable and large-scale fusion power remains a significant challenge.
Ongoing international collaboration, advancements in computing, and progress in material science will play a key role in determining whether fusion can become a practical part of the future energy mix as a potentially abundant and clean energy source.
References and Further Reading
- Temel, D. (2024). Will Nuclear Fusion Pave the Way for Widespread Sustainable Energy Use? 8(1), 9. https://doi.org/10.62802/0jvg0386
- Mohamed, M., Zakuan, N. D., Hassan, T. N. A. T., Lock, S. S. M., & Shariff, A. M. (2024). Global Development and Readiness of Nuclear Fusion Technology as the Alternative Source for Clean Energy Supply. Sustainability. https://doi.org/10.3390/su16104089
- Kumar, S., Renuka, G., Bijlwan, S., Abbas, A., Sharma, S., & Waghulde, K. B. (2024). Nuclear Fusion Reactors: Challenges and Potential as a Future Energy Source. E3S Web of Conferences, 540, 13014. https://doi.org/10.1051/e3sconf/202454013014
- Hooton, B. W. (2024). Nuclear Fusion (pp. 122–130). Oxford University Press. https://doi.org/10.1093/oso/9780198902652.003.0012
- Quadling, A. G., Lee, W. E., & Astbury, J. (2022). Materials challenges for successful roll-out of commercial fusion reactors. 4(3), 030401. https://doi.org/10.1088/2515-7655/ac73b2
- Cohen-Tanugi, D., Stapelberg, M., Short, M. P., Ferry, S., Whyte, D. G., Hartwig, Z., & Buonassisi, T. (2023). Materials for Fusion Energy: Key Challenges and Guiding Principles for Developing Under High Design Uncertainty. https://doi.org/10.48550/arxiv.2311.12187
- Staniec, P. A., Castillo, I., Suppiah, S., Boniface, H., Ryland, D. K., Brennan, D., & Lawless, R. (2023). Tritium Opportunities and Challenges for Fusion Developments Worldwide—CNL and UKAEA View. Fusion Science and Technology, 1–9. https://doi.org/10.1080/15361055.2023.2210277
- Ongena, J., Koch, R., Wolf, R., & Zohm, H. (2016). Magnetic-confinement fusion. Nature Physics, 12(5), 398–410. https://doi.org/10.1038/NPHYS3745
- Haack, J. (2024). Superconductivity for Nuclear Fusion: Past, Present, and Future. Arabian Journal for Science and Engineering. https://doi.org/10.1007/s13369-024-08720-4
- Wagner, F. (2013). Physics of magnetic confinement fusion. 54(54), 01007. https://doi.org/10.1051/EPJCONF/20135401007
- Zheng, L. (2022, January 18). Quasisymmetric stellarators. Physics, 15, 5. https://physics.aps.org/articles/v15/5
- Song, Y., Zou, X. L., Gong, X., Becoulet, M., Buttery, R. J., Bonoli, P. T., Hoang, T., Maingi, R., Qian, J., Zhong, X., Liu, A. D., Li, E., Ding, R., Huang, J., Zhang, Q., Liu, H., Wang, L., Zhang, L., Li, G., … Li, J. (2023). Realization of thousand-second improved confinement plasma with Super I-mode in Tokamak EAST. Science Advances, 9(1). https://doi.org/10.1126/sciadv.abq5273
- Ma, T. (2023). Creating a star on earth, ignition, and where lasers are taking us in the future of fusion energy. https://doi.org/10.2351/7.0001494
- Burby, J. W., Maldonado, I. A., Ruth, M., Messenger, D. A., & Carbajal, L. (2025). Nonperturbative guiding center model for magnetized plasmas. Physical Review Letters, 134(17), 175101. https://doi.org/10.1103/PhysRevLett.134.175101
- Airhart, M. (2025, May 10). Scientists crack 70-year fusion puzzle, paving way for clean energy. SciTechDaily. https://scitechdaily.com/scientists-crack-70-year-fusion-puzzle-paving-way-for-clean-energy/
Disclaimer: The views expressed here are those of the author expressed in their private capacity and do not necessarily represent the views of AZoM.com Limited T/A AZoNetwork the owner and operator of this website. This disclaimer forms part of the Terms and conditions of use of this website.