Continental-scale impacts on ecosystems are often not extensively recorded. This article details the use of ice core retrieved from the Monte Rosa Massif in the Swiss Alps to reveal close association among vegetation, climate, pollution, agriculture, pests, and fire in the past millennium in Europe. The article looks at Brugger, S. O et al.'s paper published in Geophysical Research Letters.
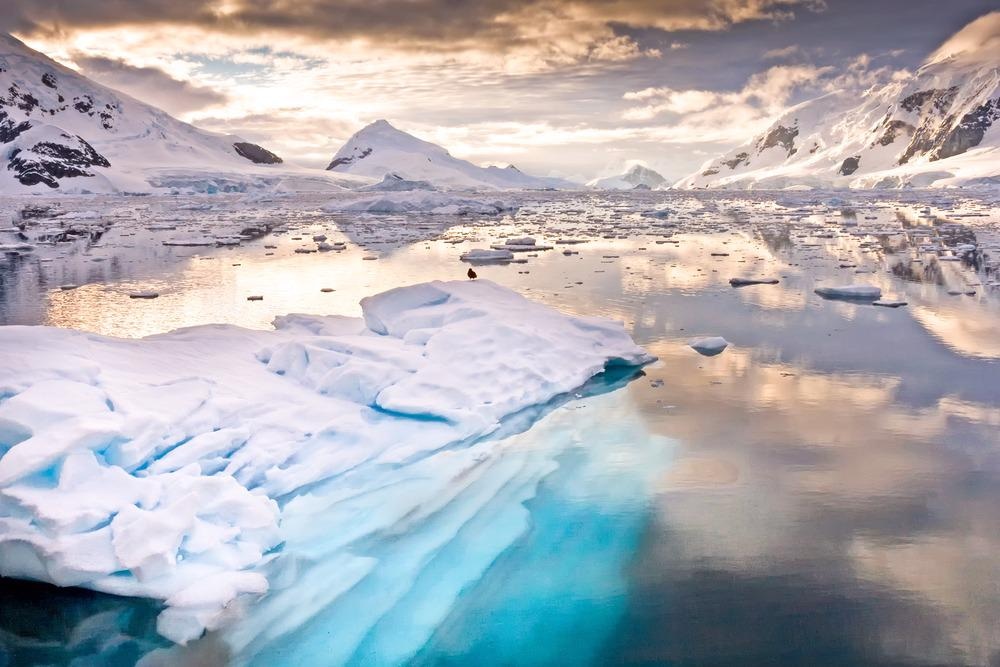
Image Credit: Wim Hoek/Shutterstock.com
Knowledge about the past ecosystem dynamics and human activities is mostly attained through narrative sources by chroniclers and diarists. However, these do not allow extrapolation to an extended region. The majority of the microfossil records from lake sediments recording ecosystem change lacks chronological precision, spatial scale, temporal resolution, and ecosystem complexity.
Recently, microfossils protected in ice cores demonstrated the strength to provide vegetation and land use-reconstructions on a large spatial scale with incomparable chronological precision, but this area is hugely unexplored.
The current research is the first of its kind analysis of a glacier ice core from the European Alps. The article analyzes how human innovation, extreme weather, crop failures, and pollution molded European ecosystems and how these societies adjusted to technological advances in a highly changing world.
The ice core was collected from Colle Gnifetti in the Monte Rosa massif, located in the heart of the European continent. The Colle Gnifetti glacier saddle is an old natural ice archive. It resisted melting due to its high elevation. Microfossils such as charcoal from burning biomass, pollen from plants, and pollution particles are preserved in the ice and transported from various areas via the atmosphere (see Figure 1).
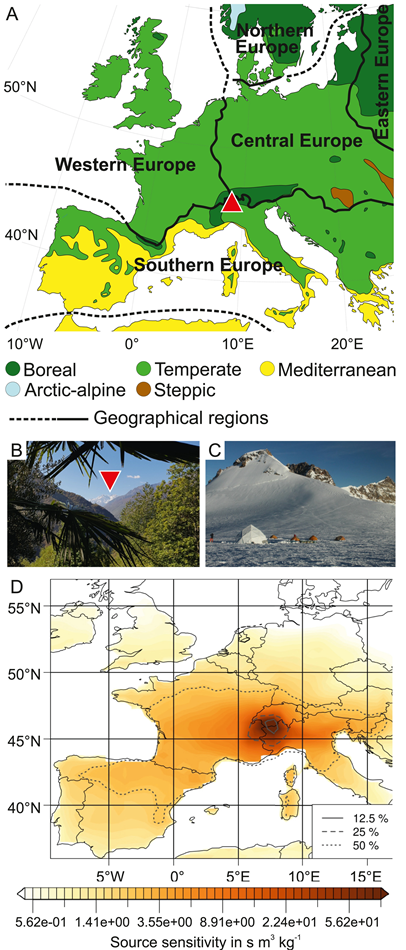
Figure 1. Study site in the Monte Rosa massif. (a) European biomes and geographical regions (Lang, 1994), as well as the study region of Monte Rosa in the European Alps (red triangle). (b) Southern view from Italy toward Monte Rosa and the Colle Gnifetti glacier saddle (red triangle; Photo: Willy Tinner). (c) Drilling camp in summer 2015 on the Colle Gnifetti glacier saddle (Photo: Michael Sigl). (d) Source sensitivity of Colle Gnifetti to different land areas are based on the atmospheric transport model FLEXPART. Source sensitivity was calculated as air mass residence time in a given grid cell divided by air density and as such is given in units (s m3 kg−1). Isolines encompass areas with the largest source sensitivity that contribute the given percentage to the total source sensitivity. Image Credit: Brugger, et al., 2021
The article utilizes the information gathered to decipher land-use dynamics and large-scale vegetation in response to environmental, biological, and societal drivers, such as an invasion, succession, disturbance, pathogen infestation, climate change, and technological innovation.
A comprehensive assessment of the major environmental and ecological forcings of European ecosystem dynamics in the past 1,000 years can be evaluated. The study also evaluates the extent of ongoing global change response processes that are rooted in the past.
Methodology
The study analyzed two ice cores from Colle Gnifetti, recovered in 2003 and 2015. The ice was dated and chronology and dating of the oldest ice core section were done by independent absolute dating techniques such as carbon (14C) isotopes and nuclear dating with lead (210Pb).
To enable microfossil analysis, the ice core was split into contiguous sections of ca. 500–1,000 g. Optical analysis of the microfossils that infer vegetation and land-use dynamics was carried out. A black carbon (BC) record and an oxygen isotopes (δ18O) record were used as an indicator of past temperatures as an additional nonspecific burning tracer.
Statistically important local pollen assemblage zones (LPAZ) were inferred with the broken stick approach. The catchment areas of the Colle Gnifetti ice core were investigated with the atmospheric transport model FLEXPART.
Results
The microfossil record showed a huge variety of pollen types and demonstrated inherent sample-to-sample noise in the pollen signal. The principal component analysis (PCA) for the pollen data shows that expansions of forest species alternated with grassland and crop plants over time.
The ice core’s stable oxygen isotope data reveal past temperature oscillations at decadal to centennial scales. This enables direct comparison and evaluation of the effect of climate dynamics and variability of the past 1,000 years across various European biomes.
The microfossil record starts in the medieval period and is indicated by the high δ18O values (see Figure 2).
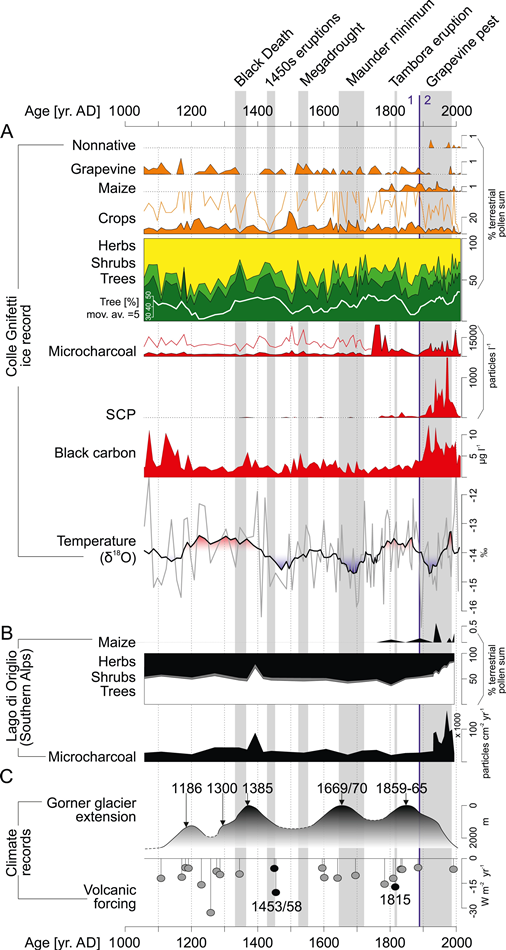
Figure 2. Comparison of the Colle Gnifetti glacier record (a) with independent data (b and c). (a) Colle Gnifetti record shows the sum of nonnative plants (Nothofagus, Pterocarya, Fallopia, Heliotropium), Vitis (grapevine), Zea mays (maize), the sum of crop, tree, shrub, and herb pollen as a proxy for vegetation and land use, microcharcoal concentrations for fire activity, spheroidal carbonaceous particles (SCP) for fossil fuel combustion, black carbon for nonspecific burning averaged to the microfossil resolution and oxygen isotopes (δ18O) for temperature averaged to microfossil resolution (gray) and as a 9-point moving average (black line). 5X exaggeration for crops and 10X exaggeration before 1750 CE for microcharcoal (red). White curve in the pollen summary graph shows 5-point moving average for tree pollen percentages. (b) Vegetation and fire reconstruction from Lago di Origlio, south of the Alps (Tinner et al., 2005). (c) Gorner glacier advances (low values) and retreats (high values) ca. 5 km northwest from Colle Gnifetti (Holzhauser et al., 2005) and cumulative global volcanic aerosol forcing (Sigl et al., 2015). Gray boxes indicate palynological signals during the Black Death, volcanic eruptions 1453/1458 CE (1450s eruptions), “Megadrought,” Maunder minimum, Tambora eruption, and the grapevine pest Phylloxera. Blue vertical line delimits statistically significant local pollen assemblage zones (LPAZ 1 and 2). Vertical dashed lines delimit 200-year intervals. Image Credit: Brugger, et al., 2021
The data revealed that around 1300 CE, the LIA cooling reflected with lower values and from 1400 to 1850 CE it resulted in the decline of crop cultivation and pastoral activities. Population decline led to the demand for grain and marginal land was converted to permanent pasture or the forest (see Figures 2 and 3).
With various other findings of major volcanic eruptions, the study also identified other preindustrial fire episodes in the ice core during the medieval period.
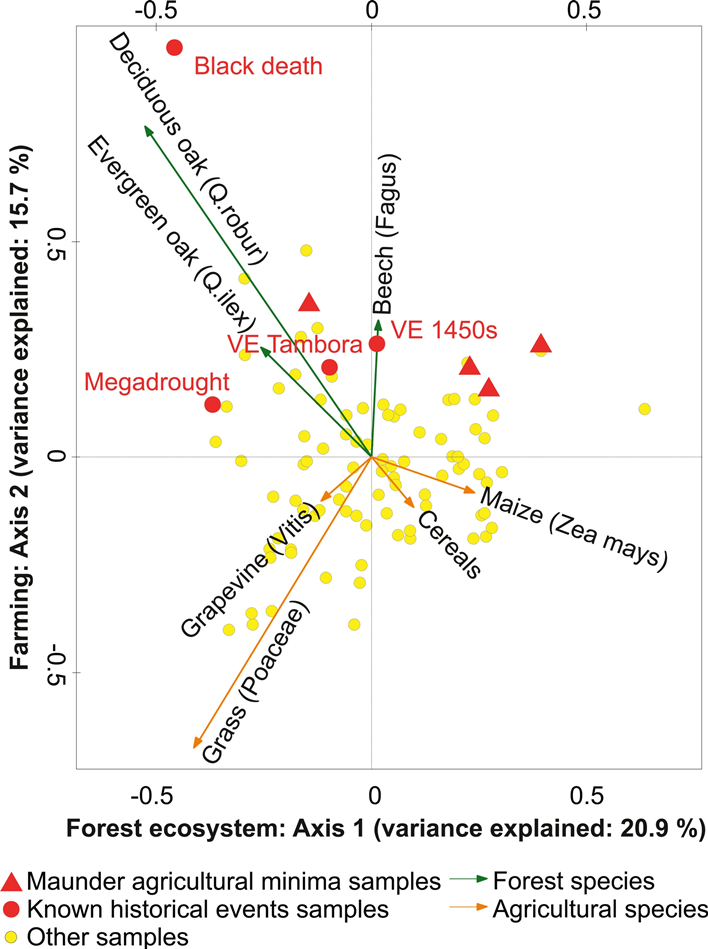
Figure 3. Sample scores for principal component analysis (PCA) for the ice pollen record. PCA based on percentages of the pollen sum. Sample scores of the following historical events are highlighted: Black Death CE 1347–1352, the volcanic eruptions CE 1453/1458 (VE 1450s), the megadrought CE 1540, volcanic eruption (VE) Tambora CE 1815. Selected taxa scores indicate the importance of farming vs. forest over the period recorded by the ice core: temperate beech oak forests (Fagus and Quercus robur), Mediterranean evergreen oak forest (Quercus ilex), crop cultivation (Cerealia, Vitis, Zea mays), and open landscape (Poaceae). Image Credit: Brugger, et al., 2021
The ice record became much more complex after 1750 CE, with the huge energy demands reflected in the Colle Gnifetti microfossil record. The introduction of many productive and resilient new crops such as maize was noted. The ice core also preserved the devastating Tambora eruption. However, the study failed to detect Solanum tuberosum (potato). It has a weak pollen dispersal, revealing the limitations.
The ice core also provides evidence of fire signals and large-scale deforestation. The spectacular shift in pollen assemblage indicates the exchange of goods over large distances.
The data obtained revealed the scale to which preindustrial societies in Europe were impacted by climatic events and epidemics.
Eventually, the impacts from industrial land use and amplified energy consumption led to unsustainable resource exploitation and these were confirmed due to tracers such as sulfate (SO4), ammonium (NH4), lead (Pb), nitrate (NO3), other heavy metals, polycyclic aromatic hydrocarbons, and radioactive isotopes in the ice core.
Conclusion
Brugger, S. O. et al.'s provides a microfossil-inferred view and reveals that acclimatization and innovation were vital in breaking the historical cycle of societal expansion. With continued acclimatizations and technological innovations, society can identify sustainable land use approaches to decrease climate warming and the threats linked to them.
Journal Reference:
Brugger, S. O., Schwikowski, M., Gobet, E., Schwörer, C., Rohr, C., Sigl, M., Henne, S., Pfister, C., Jenk, T. M., Henne, P. D., Tinner, W. (2021) Alpine Glacier Reveals Ecosystem Impacts of Europe’s Prosperity and Peril Over the Last Millennium. Geophysical Research Letters. Available online: https://agupubs.onlinelibrary.wiley.com/doi/10.1029/2021GL095039.
References and Further Reading
- Aberth, J (2005) The Black Death: The Great Mortality of 1348–1350 – A Brief History with Documents. pp. 0–200.
- Alfani, G & Percoco, M (2019) Plague and long-term development: The lasting effects of the 1629–30 epidemic on the Italian cities. The Economic History Review, 72(4), pp. 1175–1201. doi.org/10.1111/ehr.12652.
- Barbante, C., et al. (2014) Historical Record of European Emissions of Heavy Metals to the Atmosphere Since the 1650s from Alpine Snow/Ice Cores Drilled near Monte Rosa. Environmental Science & Technology, 38(15), pp. 4085–4090. doi.org/10.1021/es049759r.
- Benedictow, O J & Benedictow, O L (2004) The Black Death, 1346–1353: The complete history. Boydell & Brewer: Woodbridge; pp. 0–433.
- Bennett, K D (1996) Determination of the number of zones in a biostratigraphical sequence. New Phytologist, 132(1), pp. 155–170. doi.org/10.1111/j.1469-8137.1996.tb04521.x.
- Birks, H J B & Gordon, A (1985) Numerical methods in Quaternary pollen analysis. Geoarchaeology, pp. 0–377. doi.org/10.1002/gea.3340010406.
- Bohleber, P., et al. (2018) Temperature and mineral dust variability recorded in two low-accumulation Alpine ice cores over the last millennium. Climate of the Past, 14(1), pp. 21–37. doi.org/10.5194/cp-14-21-2018.
- Brönnimann, S., et al. (2018) Archives of nature and archives of societies. In: White, S., et al. (Eds), The Palgrave handbook of climate history; pp. 27–36.
- Brugger, S. O., et al. (2019) Palynological insights into global change impacts on Arctic vegetation, fire, and pollution recorded in Central Greenland ice. The Holocene, 29(7), pp. 1189–1197. doi.org/10.1177/0959683619838039.
- Brugger, S. O., et al. (2019) Tropical Andean glacier reveals colonial legacy in modern mountain ecosystems. Quaternary Science Reviews, 220, pp. 1–13. doi.org/10.1016/j.quascirev.2019.06.032.
- Brugger, S. O., et al. (2018) A quantitative comparison of microfossil extraction methods from ice cores. Journal of Glaciology, 64(245), pp. 432–442. doi.org/10.1017/jog.2018.31.
- Camenisch, C., et al. (2018). Two decades of crisis: Famine and dearth during the 1480s and 1490s in Western and Central Europe. In: Famines during the “Little Ice Age” (1300–1800). Socionatural entanglements in Premodern Societies, pp. 69–90. doi.org/10.1007/978-3-319-54337-6_4.
- Campbell, B M S (2016) The great transition: Climate, disease and society in the late-medieval World. Cambridge University Press; pp. 0–490.
- Colombaroli, D., et al. (2010) Species responses to fire, climate, and human impact at tree line in the Alps as evidenced by palaeo-environmental records and a dynamic simulation model. Journal of Ecology, 98(6), pp. 1346–1357. doi.org/10.1111/j.1365-2745.2010.01723.x.
- Conedera, M., et al. (2004) The cultivation of Castanea sativa (Mill.) in Europe, from its origin to its diffusion on a continental scale. Vegetation History and Archaeobotany, 13(3), pp. 161–179. doi.org/10.1007/s00334-004-0038-7.
- Cook, E. R., et al. (2015) Old World megadroughts and pluvials during the common era. Science Advances, 1(10), p. e1500561. doi.org/10.1126/sciadv.1500561.
- Crosby, A W (2003). The Columbian exchange: Biological and cultural consequences of 1492. Vol. 2, pp. 0–312.
- Curtis, D R (2016) Was plague an exclusively urban phenomenon? Plague mortality in the 17th century low countries. Journal of Interdisciplinary History, 47(2), pp. 139–170. doi.org/10.1162/JINH_a_00975.
- Daux, V., et al. (2012) An open-access database of grape harvest dates for climate research: Data description and quality assessment. Climate of the Past, 8(5), pp. 1403–1418. doi.org/10.5194/cp-8-1403-2012.
- Davis, M A (2003) Biotic globalization: Does competition from introduced species threaten biodiversity? BioScience, 53(5), pp. 481–489. doi.org/10.1641/0006-3568(2003)053[0481:BGDCFI]2.0.CO;2.
- Döscher, A., et al. (1995) A 130 yr deposition record of sulfate, nitrate, and chloride from a high-alpine glacier. Water, Air, and Soil Pollution, 85(2), pp. 603–609. doi.org/10.1007/BF00476895.
- Döscher, A., et al. (1996) A historical record of ammonium concentrations from a glacier in the Alps. Geophysical Research Letters, 23(20), pp. 2741–2744. doi.org/10.1029/96GL02615.
- Eichler, A., et al. (2017) Ice-core evidence of earliest extensive copper metallurgy in the Andes 2700 yr ago. Scientific Reports, 7(1), pp. 1–11. doi.org/10.1038/srep41855.
- Esper, J., et al. (2017) Northern Hemisphere temperature anomalies during the 1450s period of ambiguous volcanic forcing. Bulletin of Volcanology, 79(41), pp. 3–9. doi.org/10.1007/s00445-017-1125-9.
- Fischer, H., et al. (2018) Palaeoclimate constraints on the impact of 2°C anthropogenic warming and beyond. Nature Geoscience, 11, pp. 474–485. doi.org/10.1038/s41561-018-0146-0.
- Gabrieli, J & Barbante, C (2014) The Alps in the age of the Anthropocene: The impact of human activities on the cryosphere recorded in the Colle Gnifetti glacier. Rendiconti Lincei, 25(1), pp. 71–83. doi.org/10.1007/s12210-014-0292-2.
- Gabrieli, J., et al. (2011) Contamination of Alpine snow and ice at Colle Gnifetti, Swiss/Italian Alps, from nuclear weapons tests. Atmospheric Environment, 45(3), pp. 587–593. doi.org/10.1016/j.atmosenv.2010.10.039.
- Gabrieli, J., et al. (2010) Post 17th century changes of European PAH emissions recorded in high-altitude alpine snow and ice. Environmental Science & Technology, 44(9), pp. 3260–3266. doi.org/10.1021/es903365s.
- Gale, G (2011) Dying on the Vine. How Phylloxera transformed wine. doi.org/10.1525/9780520948853.
- Gobet, E., et al. (2003) Holocene vegetation history of the Upper Engadine (Swiss Alps): The role of man and fire. Vegetation History and Archaeobotany, 12(3), pp. 143–163. doi.org/10.1007/s00334-003-0017-4.
- Hoffmann, R C (2014) An environmental history of medieval Europe. Cambridge University Press; pp. 0–428.
- Holzhauser, H., et al. (2005) Glacier and lake-level variations in west-central Europe over the last 3,500 yr. The Holocene, 15(6), pp. 789–801. doi.org/10.1191/0959683605hl853ra.
- Jenk, T. M., et al. (2009) A novel radiocarbon dating technique applied to an ice core from the Alps indicating late Pleistocene ages. Journal of Geophysical Research, 114, p. D14305. doi.org/10.1029/2009JD011860.
- Kidwell, S M (2015) Biology in the Anthropocene: Challenges and insights from young fossil records. Proceedings of the National Academy of Sciences, 112(16), pp. 4922–4929. doi.org/10.1073/pnas.1403660112.
- Lang, G (1994). Quartäre vegetationsgeschichte Europas: Methoden und Ergebnisse. Gustav Fischer; pp. 0–462.
- Lemmen, C & Wirtz, K W (2014) On the sensitivity of the simulated European Neolithic transition to climate extremes. Journal of Archaeological Science, 51, pp. 65–72. doi.org/10.1016/j.jas.2012.10.023
- Liu, K. B., et al. (2015) Ice-core pollen record of climatic changes in the central Andes during the last 400 yr. Quaternary Research, 64(2), pp. 272–278. doi.org/10.1016/j.yqres.2005.06.001.
- Loveluck, C. P., et al. 92018) Alpine ice-core evidence for the transformation of the European monetary system, CE 640–670. Antiquity, 92(366), pp. 1571–1585. doi.org/10.15184/aqy.2018.110.
- Luterbacher, J & Pfister, C (2015) The year without a summer. Nature Geoscience, 8, pp. 246–248. doi.org/10.1038/ngeo2404.
- MacDonald, D., et al. (2000) Agricultural abandonment in mountain areas of Europe: Environmental consequences and policy response. Journal of Environmental Management, 59(1), pp. 47–69. doi.org/10.1006/jema.1999.0335.
- Mack, R N & Lonsdale, W M (2001) Humans as global plant dispersers: Getting more than we bargained for: Current introductions of species for aesthetic purposes present the largest single challenge for predicting which plant immigrants will become future pests. BioScience, 51(2), pp. 95–102. doi.org/10.1641/0006-3568(2001)051[0095:HAGPDG]2.0.CO;2.
- Malanima, P (2009) Pre-modern European economy. One thousand years (10th–19th centuries). Brill; pp. 0–428.
- Malanima, P (2016) Energy consumption in England and Italy, 1560–1913. Two pathways toward energy transition. The Economic History Review, 69(1), pp. 78–103. doi.org/10.1111/ehr.12109.
- McAleer, J (2016) “A young slip of botany”: Botanical networks, the South Atlantic, and Britain’s maritime worlds, c. 1790–1810. Journal of Global History, 11(1), pp. 24–43. doi.org/10.1017/S1740022815000339.
- Montanari, M. (1996) The culture of food (The making of Europe). Blackwell; pp. 0–228.
- More, A. F., et al. (2017) Next-generation ice core technology reveals true minimum natural levels of lead (Pb) in the atmosphere: Insights from the Black Death. GeoHealth, 1(4), pp. 211–219. doi.org/10.1002/2017GH000064.
- More, A. F., et al. (2018) The role of historical context in understanding past climate, pollution and health data in trans-disciplinary studies: Reply to comments on More et al., 2017. GeoHealth, 2(5), pp. 162–170. doi.org/10.1029/2017GH000121.
- Niemann, H., et al. (2013) Late Holocene human impact and environmental change inferred from a multi-proxy lake sediment record in the Loja region, southeastern Ecuador. Quaternary International, 308, pp. 253–264. doi.org/10.1016/j.quaint.2013.03.017.
- Pfister, C (1990). The early loss of ecological stability in an agrarian region. Nitrogen, energy, and the take-off of «solar» agriculture in the Canton of Bern. In: Brimblecombe, P & Pfister C (Eds), The silent countdown, pp. 37–55. doi.org/10.1007/978-3-642-75159-2_4.
- Pfister, C., et al. (2015) Tree-rings and people-different views on the 1540 megadrought. Reply to Büntgen et al., 2015. Climate Change, 131(2), pp. 191–198. doi.org/10.1007/s10584-015-1429-8.
- Pisso, I., et al. (2019) The Lagrangian particle dispersion model FLEXPART version 10.4. Geoscientific Model Development, 12, pp. 4955–4997. doi.org/10.5194/gmd-12-4955-2019.
- Radkau, J (2012). Wood: A history. Polity; pp. 0–352.
- Rey, F., et al. (2019) Causes and mechanisms of synchronous succession trajectories in primeval Central European mixed Fagus sylvatica forests. Journal of Ecology, 107(3), pp. 1392–1408.
- Roberts, N., et al. (2018) Europe’s lost forests: A pollen-based synthesis for the last 11,000 yr. Scientific Reports, 8(1), pp. 1–8. doi.org/10.1038/s41598-017-18646-7.
- Schwikowski, M., et al. (2004) Post-17th century changes of European lead emissions recorded in high-altitude alpine snow and ice. Environmental Science & Technology, 38(4), pp. 957–964. doi.org/10.1021/es034715o.
- Schwikowski, M., et al. (1999) Anthropogenic versus natural sources of atmospheric sulfate from an Alpine ice core. Tellus B: Chemical and Physical Meteorology, 51(5), pp. 938–951. doi.org/10.3402/tellusb.v51i5.16506.
- Schwörer, C., et al. (2014) Holocene climate, fire and vegetation dynamics at the treeline in the Northwestern Swiss Alps. Vegetation History and Archaeobotany, 23(5), pp. 479–496. doi.org/10.1007/s00334-013-0411-5.
- Sigl, M., et al. (2018) 19th century glacier retreat in the Alps preceded the emergence of industrial black carbon deposition on high-alpine glaciers. The Cryosphere, 12(10), pp. 3311–3331. doi.org/10.5194/tc-12-3311-2018.
- Sigl, M., et al. (2015) Timing and climate forcing of volcanic eruptions for the past 2,500 yr. Nature, 523(7562), pp. 543–549. doi.org/10.1038/nature14565.
- Simberloff, D., et al. (2010) Spread and impact of introduced conifers in South America: Lessons from other Southern Hemisphere regions. Australian Ecology, 35(5), pp. 489–504. doi.org/10.1111/j.1442-9993.2009.02058.x.
- Smil, V (2017) Energy transitions, global and national perspectives. Praeger.
- Sombart, W (1919) Der moderne Kapitalismus. Historisch-systematische Darstellung des gesamteuropäischen Wirtschaftslebens von seinen Anfängen bis zur Gegenwart. Erster Band: Die vorkapitalistische Wirtschaft. Duncker und Humblot.
- Summermatter, S (2012) Die Prävention von Überschwemmungen durch das politische System der Schweiz von 1848 bis 1991 (Doctoral dissertation). Institute of History, University of Bern.
- Ter Braak, C J & Prentice, I C (1988) A theory of gradient analysis. Advances in Ecological Research, 18, pp. 271–317. doi.org/10.1016/S0065-2504(08)60183-X.
- Tinner, W., et al. (2005) Fire ecology North and South of the Alps since the last ice age. The Holocene, 15(8), pp. 1214–1226. doi.org/10.1191/0959683605hl892rp.
- Tinner, W., et al. (2003) Climatic change and contemporaneous land-use phases North and South of the Alps 2300 BCE to 800 CE. Quaternary Science Reviews, 22(14), pp. 1447–1460. doi.org/10.1016/S0277-3791(03)00083-0.
- van der Knaap, W. O., et al. (2000) Palynostratigraphy of the last centuries in Switzerland based on 23 lake and mire deposits: Chronostratigraphic pollen markers, regional patterns, and local histories. Reviews of Paleobotany and Palynology, 108(1–2), pp. 85–142. doi.org/10.1016/S0034-6667(99)00035-4.
- Vannière, B., et al. (2008) Climate versus human-driven fire regimes in Mediterranean landscapes: The Holocene record of Lago dell’Accesa (Tuscany, Italy). Quaternary Science Reviews, 27(11–12), pp. 1181–1196. doi.org/10.1016/j.quascirev.2008.02.011.
- Vasil’chuk, A C & Vasil’chuk, Y K (2020) Pollen as a potential indicator of the origin of massive ice in northwest Siberia. Permafrost and Periglacial Processes, 32, pp. 1–367. doi.org/10.1002/ppp.2095.
- Wetter, O., et al. (2014) The year-long unprecedented European heat and drought of 1540—A worst case. Climatic Change, 125(3), pp. 349–363. doi.org/10.1007/s10584-014-1184-2.